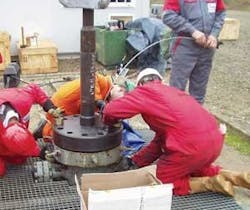
Optical fibers are useful in “down-hole” energy applications (which include oil, gas, and geothermal wells), serving either as data conduits or sensors. For example, point sensors such as fiber Bragg gratings and Fabry-Perot interferometers monitor the temperature, pressure, and flow properties of multiphase fluids, with their signals sent to the surface via fiber. In another approach, an optical fiber can be used as a distributed temperature and pressure sensor, by which it is possible to obtain temperature and pressure profiles along the entire length of the well at resolutions approaching several meters. In this case, backscattered light from the fiber (which is influenced by local temperature or pressure by either the Raman or Brillouin effect) is analyzed using time-domain reflectometry, which pinpoints the spatial position of the scattering element.
Such fibers, however, can become victims of the very high pressures and temperatures they are intended to monitor. For example, the high hydrogen partial pressure found in many down-hole environments results in the diffusion of molecular hydrogen (H2) into the core of the fiber, darkening the fiber in a matter of days, especially within the 800- to 1700-nm wavelength window of interest. To combat this problem, engineers at Verrillon (North Grafton, MA) have optimized a carbon coating that blocks the entry of H2; the coating extends fiber lifetime from days to tens of years, according to Imtiaz Majid, product manager.
The resulting harsh-environment fibers, which can be either single- or multimode, have numerical apertures ranging from 0.07 to 0.3, core sizes ranging from several to hundreds of microns, and clad sizes that range from tens to hundreds of microns, according to Majid. The carbon coating, which is applied in a chemical-vapor-deposition process onto fibers as long as 15 km, can be combined with other coatings for structural protection. Because temperatures in down-hole environments can approach 300°C, ordinary telecommunications-grade acrylate coatings just won’t do; instead, heat-resistant coating such as polyimide must be used.
“The development of any carbon coating to be applied on a pristine glass surface is a highly nontrivial process,” says Majid. “The carbon can be deposited on the glass surface in several forms: amorphous, graphitic, or diamondlike. As well, great care must be taken in designing the details of the deposition chamber. It is crucial to design the deposition chamber to ensure uniform deposition of the carbon, to maintain uniform deposition temperatures with tight feedback control, and to have extremely good control over the flow of gaseous mixtures into the deposition region.” One of the major issues associated with carbon deposition is related to the slightly reduced mean strength of the fiber, notes Majid. “This effect is always present in carbon-coated glass optical fibers; however, it is possible to reduce the magnitude of this effect by ensuring an ultraclean deposition environment and using highly optimized deposition temperatures,” he says.
The Verrillon engineers improved the structure of the deposited carbon to ensure the highest degree of hermeticity with respect to H2 without significantly affecting other properties of the fiber (such as mechanical strength). Majid notes that the carbon coating is also an effective barrier to moisture (as evidenced by a measured stress-corrosion parameter “n value” of greater than 100), as well as to acids. Because moisture is known to be an extremely effective agent in reducing the mechanical strength of the fiber, the carbon helps in maintaining the structural integrity of the fiber as well. In one experiment, fibers with and without carbon coatings were exposed to a mixture of hydrofluoric acid, ammonium fluoride, and water vapor. The carbon-coated fibers exhibited no shift in mean strength, while the non-carbon-coated fibers disintegrated when handled.
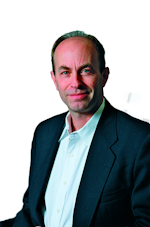
John Wallace | Senior Technical Editor (1998-2022)
John Wallace was with Laser Focus World for nearly 25 years, retiring in late June 2022. He obtained a bachelor's degree in mechanical engineering and physics at Rutgers University and a master's in optical engineering at the University of Rochester. Before becoming an editor, John worked as an engineer at RCA, Exxon, Eastman Kodak, and GCA Corporation.