Photonic Frontiers: Fiber Optics: Looking Back/Looking Forward: Fiber optics—booms, one spectacular bust, and a bright future
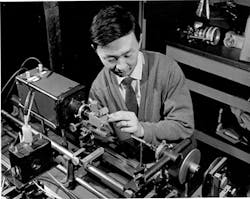
Fiber optics were invented for medical and military imaging more than half a century ago, and are still used for imaging, light guiding, and other applications. But their major use is in telecommunications, where the invention of the laser led to the discovery of a little problem with the air. Fog, haze, and weather could block the beam, and air turbulence could divert or distort it. Bell Labs eventually turned to hollow light pipes for terrestrial communications, modeled on hollow millimeter waveguides being developed for 60 GHz microwaves.
Laser links seemed more promising for space. Our August 15, 1965 issue (pp. 4–5) described plans by RCA's Astro-Electronics division for a 10 Mbit laser link to support the first manned mission to Mars, which they then thought might be launched by 1980.
A story in our April 1, 1966 issue (pp. 3–4) would prove far more prophetic. We reported that Charles K. Kao of ITT Standard Telecommunications Laboratories (Harlow, Essex, England) had told a London meeting how single-mode optical fibers could transmit light signals with gigahertz bandwidth. Kao had asked how clear glass could be made, and Harold Rawson of Sheffield University (Sheffield, South Yorkshire, England) had said the attenuation could be reduced below 20 dB/km, enough for signals to go several kilometers between telephone switching offices. Figure 1 shows Kao measuring the transparency of fused silica.
An unexpected breakthrough
Imaging and light piping were the biggest fiber-optic markets listed in our July 1969 issue by Narinder S. Kapany of Optics Technology (Palo Alto, CA). He put annual sales at $7 million for fused fiber optics for military vision systems, $2 million for flexible aligned bundles such as endoscopes, $2 million for unaligned fiber assemblies, and $1 million for special unaligned fiber assemblies.Robert Maurer, Donald Keck, and Peter Schultz scored the first of two crucial breakthroughs at the Corning Glass Works (Corning, NY) in 1970 by making a fused silica fiber with loss of only 17 dB/km. Their second came in 1972, producing fibers far more durable with loss of about 2 dB/km. That brought rapid progress. In our September 1974 issue (pp. 36–40), Forrest M. Mims wrote "the immediate commercial future for laser communications seems to lie in optical-fiber links for telephone, video and computer systems within a city or between adjacent communities." Military agencies also were big supporters, seeking small size and light weight as well as immunity from electromagnetic interference.
A November 1975 editorial (p. 6) noted ongoing tests by AT&T and General Telephone and Electronics. After exhaustive tests, AT&T conservatively designed a standard system to carry 45 Mbit/s from 820 nm diode lasers through several 10 km of multimode fiber. In March 1977 (p. 26), we reported AT&T would install two short 24-fiber cables to carry live telephone calls and Bell's Picturephone video meeting service in Chicago. In May 1977 (p. 34), we reported GTE plans for a modest 6.3 Mbit/s test, which they had up and running in Long Beach, CA, on April 22 before our story was printed. The AT&T cables started carrying traffic on May 11, our July 1977 issue reported (p. 70). Our January 1978 cover (see Fig. 2) showed a crew installing the Chicago system. In July 1978, we reported the Bell System would put similar fiber-optic links into regular telephone service "before 1981."
Visionaries and big systems
Others had bigger visions for fiber. In 1972, GTE Labs engineer John Fulenwider proposed using fibers instead of coaxial cable in a "wired city" project. In November 1976 (p. 16), we reported Japanese plans for fiber links between some 300 homes and a centralized computer information service. Masahiro Kawahata, managing director of the Visual Information System Development Association, wanted "to explore an ideal information transmission system for a modern society." We described the first eight months of the Hi-OVIS system in January 1980 (pp. 58–60). Users were happy and children loved video on demand, but serving 158 homes in Higashi Ikoma cost some $40 million (see Fig. 3).Fiber technology changed as Japanese researchers opened longer-wavelength windows. In early 1976, Masaharu Horiguchi of Nippon Telegraph and Telephone (Ibaraki, Japan) and Hiroshi Osanai of Fujikura Cable measured 0.47 dB/km loss at 1.3 μm and later found even lower loss at 1.51 μm. In August 1980, we reported NTT had used 1.3 μm to send 100 Mbit/s a record 30 km and 1.6 Gbit/s for 13.3 km (p. 50).
In March 1980 (pp. 60–62), we reported AT&T plans for a $79 million system of up to 144 multimode fibers, each carrying 44.7 Mbit/s at 820 nm, along the Northeast Corridor from Boston to Washington, with repeaters every 6.4 km. The plan evolved over time, adding additional wavelengths at 875 and 1300 nm, and raising data rates to 90 Mbit/s.
Our May 1980 (pp. 68–69) issue reported Bell plans for the world's first transatlantic fiber-optic cable, transmitting 274 Mbit/s through two pairs of single-mode fibers. "The next generation of coaxial cables will not be developed because of fibers," said Peter K. Runge of Bell Labs (Holmdel, NJ). Fiber transmission at 1.3 μm allowed 30–35 km repeater spacing, more than three times that of the last coaxial submarine cable. The target for completion was 1988.
Rapid advances in single-mode technology led NTT to plan a field trial of 400 Mbit/s single-mode transmission at 1.3 μm starting in mid-1982, we reported in July 1981 (p. 93). With fiber loss of 0.49 dB/km, they expected repeater spacing of 20 km, with commercial service to start in 1983. The turning point for singlemode in the U.S. came at the end of 1982, when MCI picked it for national backbone networks carrying 400 Mbit/s. By the time the AT&T's pioneering multimode wavelength-division multiplexing (WDM) system was completed in 1984, it was essentially obsolete.
Fiber amplifiers and WDM
Another fiber-optic revolution began later in the 1980s with David Payne's development of the erbium-doped fiber amplifier (EDFA) at the University of Southampton (Southampton, Hampshire, England). Early long-haul networks needed cumbersome electro-optic repeaters, so all-optical amplifiers were the answer. Even better, EDFAs were in the 1550 nm band, where fiber attenuation was lowest. In February 1989 (p. 129), we reported 8 dB gain in an EDFA amplifying 140 Mbit/s at British Telecom Research Laboratories (Ipswich, Suffolk, England). In July 1990, we reported the first commercial EDFA from a BT joint venture with DuPont, with 15 dB of gain across a 25 nm band (see Fig. 4).The EDFA's wide bandwidth revived interest in wavelength-division multiplexing, although its gain had to be flattened—but that soon came. In December 1994 (p. 83), we reported Andrew Chraplyvy at Bell Labs (Holmdel, NJ) had transmitted 16 2.5 Gbit/s channels through a 1420 km length of fiber containing 14 EDFAs.
Fiber capacity grew in phase with Internet traffic, creating a boom market. In April 1999 (p. 51), we reported a record 11,300 attendees and crowded exhibits at the Optical Fiber Communications conference in San Diego. KDD R&D Laboratories (Saitama, Japan) pointed the way to future systems by sending 50 10 Gbit/s optical channels spaced at 50 GHz intervals through 4000 km to achieve a record bit-rate-distance product of 2.15 pbit-km/s. That data rate and channel spacing would become standard for backbone systems for many years to come. The business was booming so much that in 1999, Laser Focus World spun off a separate magazine called WDM Solutions to cover fiber-optic components.
The bubble
OFC took "a quantum leap" in March 2000, with attendance jumping some 50% to 17,378, we reported in May 2000 (p. 111). The NASDAQ peaked at 5000 during the conference, the press room was crawling with market analysts, and the registration line wound around the Baltimore Convention Center. Money and technology were in the air. CoreTek (Wilmington, MA) reported an optically pumped tunable VCSEL in the postdeadline session (see Fig. 5), and Nortel quickly bought them for $1.43 billion.After OFC, dot-com failures pulled the NASDAQ down, but optics deals grew. JDS Uniphase's purchase of E-TEK Dynamics closed at $18.7 billion, we reported in August 2000 (p. 95). The biggest deal was JDSU's merger with SDL Inc., worth a staggering $41 billion on July 10, 2000. JDSU's market capitalization peaked at $181 billion in the summer, and then began slipping with the rest of the market.
Much of OFC 2001 in the Anaheim Convention Center seemed encouraging, we reported in May 2001 (p. 20). Attendance doubled to over 38,000, and exhibitors also doubled to 970 companies. A pre-show market seminar at the Disneyland Hotel displayed an industry determined to charge ahead. But stocks were down, the economy was slowing, and Corning reported its photonics growth would be less than half of earlier expectations. In retrospect, the industry had already run off the cliff and hung in mid-air with legs churning, like Wile E. Coyote waiting for the law of gravity to take hold when he looked down.
OFC attendance dropped to 32,000 the next year in Anaheim, but exhibitor count rose to 1227, we reported in May 2002. "Last year's OFC was a spectacle of venture-capital proportions, [but] this year's was nearly mundane by comparison, reflecting the growing pains the optical networking industry has endured the last 12 months." A scattering of empty booths in the exhibit hall quietly showed the toll. Stocks continued sliding, and we folded WDM Solutions into Lightwave.In retrospect, I wrote in February 2003, I was surprised how poorly we understood network traffic and capacity. The oft-repeated mantra that Internet traffic was doubling every three months came from a February 1997 press release from WorldCom. It might have been true in 1995 and 1996, said Andrew Odlyzko of the University of Minnesota, but since then it had been doubling every year (see Fig. 6). He estimated U.S. Internet in late 2002 averaged about 300 Gbit/s, less than half the capacity of a single fiber carrying 80 WDM channels at 10 Gbit/s each. Market analyst Shing Yin of RHK (San Francisco, CA) put the average only a little higher, 500 Gbit/s, at the end of 2002.
Dark fiber and new technology
The bubble left one positive legacy-huge amounts of dark fiber to foster future growth. Traffic continues to increase at healthy rates, and a new generation of coherent transmission systems began to come on line at 100 Gbit/s, I wrote in March 2010. The previous December, Verizon had turned on a 100 Gbit/s line running 893 km from Paris to Frankfurt, which used the same 50 GHz bandwidth as a 10 Gbit signal. That impressive performance came from coherent transmission, electronic dispersion compensation, phase shift keying, multiple bits per symbol, and polarization-division multiplexing.
100 Gbit/s systems now are installed on long-haul routes of 1500 to 2000 km. In February 2014, I wrote that the cutting edge had moved to optical "superchannels" carrying hundreds of gigabits per second using advanced modulation to transmit more than one bit per second per hertz of bandwidth. In a postdeadline paper at the European Conference on Optical Communications in September 2013, TE Subcom reported coming within 2.5 dB of the limit in a 9100 km experiment that reached 4.93 bits/s/Hz efficiency. Laboratory hero experiments are testing spatial-division multiplexing through multiple cores in a single fiber or multiple modes in a single core.
Two hero experiments reported at the OFC 2015 postdeadline session used few-mode cores in multimode fibers to carry over 100 channels. Jun Sakaguchi of the National Institute of Information and Communications Technology (Tokyo, Japan) and colleagues sent three modes through all 36 core in a 5.5 km fiber.1 Koji Igarashi of KDDI R&D Labs (Saitama, Japan) sent six modes through 19 cores in a 9.8 km fiber.2
That technology faces plenty of practical problems, notably fabricating and connecting the cores in multicore fibers, and effectively isolating the modes in few-mode fibers. Lighting up dark fiber may be more cost-effective for years to come. But specialized ultra-high capacity fibers may find their own niches, such as connecting datacenters.
Meanwhile, the visionaries' dreams of fiber to the home are coming true—I now have a 25 Mbit/s fiber link to my home and Verizon is offering me a deal on 50 Mbit/s. Elsewhere, Google Fiber and AT&T are offering a gigabit. We're not sure yet what to do with all that bandwidth, but a look at history says we're sure to find something.
REFERENCES
1. J. Sakaguchi et al., "Realizing a 36-core, 3-mode fiber with 108 spatial channels," Optical Fiber Communication Conference Post Deadline Papers, OSA Technical Digest (online), paper Th5C.2 (2015).
2. K. Igarashi et al., "114 space-division-multiplexed transmission over 9.8-km weakly-coupled-6-mode uncoupled-19-core fibers," Optical Fiber Communication Conference Post Deadline Papers, OSA Technical Digest (online), paper Th5C.4 (2015).
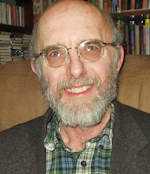
Jeff Hecht | Contributing Editor
Jeff Hecht is a regular contributing editor to Laser Focus World and has been covering the laser industry for 35 years. A prolific book author, Jeff's published works include “Understanding Fiber Optics,” “Understanding Lasers,” “The Laser Guidebook,” and “Beam Weapons: The Next Arms Race.” He also has written books on the histories of lasers and fiber optics, including “City of Light: The Story of Fiber Optics,” and “Beam: The Race to Make the Laser.” Find out more at jeffhecht.com.