Fiber for Fiber Lasers: Bismuth-doped optical fibers: Advances in an active laser media
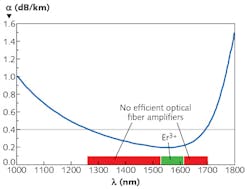
EVGENY DIANOV
Photonic materials research continues to attract great interest because of a constant need for novel lasers and optical amplifiers for many applications; in particular, for the next generation of optical fiber communication systems. And although commercial fiber-optic communication systems have per-fiber capacities of up to 10 Tbit/s and the data rate in experimental systems has reached approximately 100 Tbit/s, the demand for information in developed countries increases by 30–40% every year. This means that in 10 years, there will be a need for data transmission at petabit rates.
A number of approaches to this problem have been discussed in the literature. One possibility is to extend the spectral range of modern data transmission networks beyond the narrow (80 nm) spectral range from 1530 to 1610 nm, as defined by the gain bandwidth of erbium-doped fiber amplifiers (EDFAs). At the same time, silica-based optical fibers have low optical losses (less than 0.35 dB/km) in a considerably broader range (1300–1700 nm) and could be used for data transmission (see Fig. 1).
At present, efficient fiber lasers and optical amplifiers—necessary components of high-speed optical fiber communication systems—are missing for the 1300–1520 and 1610–1700 nm spectral ranges. The most efficient active media for near-infrared (NIR) spectral regions is rare-earth-doped fibers. However, their luminescence bands are unsuitable for creating efficient lasers and optical amplifiers in these spectral ranges of interest.
In 2001, it was discovered that bismuth (Bi)-doped aluminosilicate glass emits luminescence in an exceptionally wide region from 1000 to 1600 nm, with the luminescence bands being very broad (200–300 nm).1 While this discovery caused significant activity in the fabrication and investigation of various Bi-doped glasses, interest has steadily increased in this potentially important new active gain media since the first demonstration of a continuous-wave (CW) Bi-doped fiber laser in 2005.2
Exploring Bi-doped recipes
The first Bi-doped optical fiber was fabricated in 2005 using the modified chemical vapor deposition (MCVD) technique that is typically used for fabrication of low-loss optical fibers. The core of these early fibers consisted of Bi-doped aluminosilicate glass. Later, various types of Bi-doped optical fibers were developed, including fibers with cores consisting of Bi-doped silicon oxide and germanium oxide (SiO2 and GeO2), phosphorus blends like Bi-doped P2O5-SiO2 (phosphosilicate), and hybrid blends like Bi-doped GeO2-SiO2 (germanosilicate) and Bi-doped P2O5-GeO2-SiO2 (phosphogermanosilicate) glass.
All Bi-doped fibers have several broad absorption bands in the visible and NIR regions and the absorption spectra depend on the core glass composition. An analysis of the contour plots of the luminescence intensity for various Bi-doped fibers reveals a simple luminescence picture, for example, of Bi-doped silica fiber wherein the well-known red luminescence at 600 nm from the Bi2+ ion is visible (see Fig. 2).A NIR luminescence spectrum consists of two bands at 830 and 1430 nm for silica glass. For a Bi-doped germanate fiber, two new bands (in comparison with those of the Bi-doped silica fibers) appear at 950 and 1650 nm; in addition, the bands at 830 and 1400 nm for silica glass are also observed. Their appearance can be explained by the diffusion of a small quantity of silicon from the silica cladding into the germanate core during the fiber fabrication process.
Luminescence pictures of Bi-doped phosphosilicate fiber show a 750 nm band corresponding to Bi2+ while the bands at 830 and 1430 nm for silica are visible. There are also broad luminescence bands in the spectral region of 1100–1300 nm related to phosphorus.
The luminescence picture for Bi-doped aluminosilicate fiber shows that the band at 750 nm corresponds to Bi2+, the band at 820 nm is because of the presence of silica glass, and the three broad bands in the spectral region from 1150 to 1300 nm relate to aluminum (Al).
Taking into account the broad luminescence bands of the Bi-doped fibers (100 nm and more), one can see that the summary luminescence of the Bi-doped fibers extends from 800 to 1700 nm (through the whole spectral region). The lifetime values are between several hundred microseconds and 1000 μs for luminescence wavelengths longer than 1000 nm; for shorter wavelengths, they are 3–50 μs. A detailed discussion of the luminescence properties of Bi-doped fibers can be found in the scientific literature.3
Bi-related NIR emission
To date, the nature of Bi-related NIR-emitting centers is not clear and this circumstance makes it difficult to create efficient active media using Bi-doped glasses. Bismuth is a polyvalent element with four oxidation states: Bi5+, Bi3+, Bi2+, and Bi+. Usually Bi2O3 (oxidation state 3+) is used as a raw material for Bi-doped glass synthesis.
Two processes take place in molten Bi-doped glass: oxidation (higher valence state) and reduction (lower valence state). So the Bi ions in molten glass are in a reduction/oxidation (redox) equilibrium that depends strongly on the melting temperature, glass composition, atmosphere, and Bi concentration. These features of Bi make it notably difficult to determine its valence state and, subsequently, the exact nature of Bi-related NIR emitting centers in glass.
There are a number of hypotheses concerning the origin of these NIR centers: Bi+, Bi0, BiO, Bi2-, Bi22-, point defects, and others, but none has been directly confirmed. However, there are several experimental facts that allow one to make more definite conclusions regarding the origin of NIR luminescence in glass optical fibers.
First, it is known that Bi3+ and Bi2+ ions emit visible luminescence and no NIR luminescence. Second, the results of many experiments clearly demonstrate that NIR luminescence is observed in glasses because of the reduction of Bi3+ ions to a lower oxidation state. And finally, optically active thallium (Tl) and lead (Pb) centers in crystals have NIR luminescence bands in a range of 900–1700 nm at the excitation wavelengths from 400 to 1100 nm. It was found that the NIR emitting centers consist of definite Tl or Pb ions (or neutral atoms) and adjacent anion vacancies.4,5
Taking into account that the NIR luminescence bands of Bi-doped fibers are also located in the same spectral region and that Tl0, Pb+, and Bi2+ are isoelectronic with each other (6s26p1), there are substantial grounds to suppose that the Bi-related centers, emitting in NIR range, also consist of some Bi ions and adjacent oxygen-deficiency defects.
Our recent experiments confirmed that Bi-related active NIR emitting centers in Bi-doped germanosilicate fibers are clusters consisting of Bi ions and oxygen vacancies but not Bi ions themselves.6 And yet, the exact valence state of Bi ions still eludes us.
Creating active Bi-doped media
The first Bi-doped fiber lasers (BDFLs) were developed for a spectral region of 1140–1215 nm using Bi-doped aluminosilicate fibers. Later, BDFLs were developed for a spectral region of 1270–1800 nm using newly developed phosphogermanosilicate, germanosilicate, and silica fibers.
A 1270 nm fiber laser is of interest for many applications; in particular, for medical applications.7 In addition, the second harmonic of such a laser can substitute for a helium-neon (HeNe) gas laser. Using a 25 W, 1230 nm Raman fiber laser as a pump source, our team used a Bi-doped germanophosphosilicate fiber as a gain medium to develop this 1270 nm laser, and achieved an output power of 7.5 W.
Our team has also developed high-power, efficient BDFLs operating in the spectral region from 1390 to 1530 nm.8 A Bi-doped germanosilicate fiber with a broad luminescence band at approximately 1400 nm was used as the active medium and a phosphosilicate fiber Raman laser operated at 1340 nm was used as the pump source. At 1460 nm, the output power depends on the pump power and can reach values of up to 20 W (see Fig. 3). In this same spectral region (1400–1500 nm), an efficient Bi-doped fiber amplifier (BDFA) was constructed using the same Bi-doped germanosilicate fiber and a 65 mW commercial 1310 nm laser diode for pumping.9Recently, wavelengths well above 1500 nm were output by a Bi-doped fiber laser operating in a spectral range of 1625-1775 nm. In summary, CW BDFL spectral ranges overlap to span a broad range of operating wavelengths from 1140 to 1775 nm (see Fig. 4). A family of mode-locked BDFL has also been developed.10
Although Bi-doped fiber lasers and amplifiers are not quite as efficient as their rare-earth-doped counterparts and the scientific understanding behind the properties of Bi-related NIR emitting centers is still in its infancy, Bi-doped fiber lasers and amplifiers will continue to improve as these fabrication issues are resolved.
REFERENCES
1. Y. Fujimoto and M. Nakatsuka, Jpn. J. Appl. Phys., 40, L279–L281 (2001).
2. E. M. Dianov et al., Quant. Electron., 35, 12, 1083–1084 (2005).
3. S. V. Firstov et al., Opt. Express, 19, 20, 19551–19561 (2011).
4. M. Fockele et al., J. Phys. C Solid State Phys., 18, 1963–1974 (1985).
5. M. Fockele et al., J. Phys. Condens. Matter, 1, 13–26 (1989).
6. S. Firstov et al., Opt. Lett., 39, 24, 6927–6930 (2014).
7. A. A. Krasnovsky et al., Biochemistry Moscow+, 68, 9, 963–966 (2003).
8. A. V. Shubin et al., Opt. Lett., 37, 13, 2589–2591 (2012).
9. M. A. Melkumov et al., Opt. Lett., 36, 13, 2408–2410 (2011).
10. T. Noronen et al., Opt. Lett., 40, 2217–2220 (2015).
Evgeny Dianov is scientific director of the Fiber Optics Research Center of the Russian Academy of Sciences (FORC RAS), Moscow, Russia; e-mail: [email protected]; www.fibopt.ru.