PHOTONIC FRONTIERS: ALKALI-VAPOR LASERS: Diode pumping enables a new approach to alkali-vapor lasers
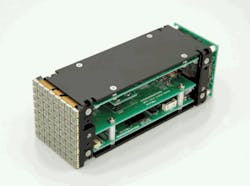
Optical pumping of alkali-metal vapors was among the first laser concepts explored in the late 1950s. Their physics were attractively simple because they have only a single valence electron in their outer shells. However, good pump sources were sorely lacking and the reactivity of alkali metals made experiments difficult. Although Paul Rabinowitz, Steve Jacobs, and Gordon Gould demonstrated an optically pumped 7.2 μm cesium laser in 1962, it found no applications and became a footnote in laser history.
Now diode pumping has revived alkali lasers. Bill Krupke, principal of WFK Lasers (Pleasanton, CA), turned to alkali vapors to get around problems of heat dissipation that limited the beam quality of kilowatt-class industrial solid-state lasers. In 2003, he reported that commercially available near-infrared laser diodes could pump alkali vapors extremely efficiently, potentially producing beams of higher quality than those available from solid-state lasers by easing thermal problems.1 Since then, diode pumping has been demonstrated for potassium, rubidium, and cesium vapor lasers, and powers have been climbing.
Alkali-vapor basics
Atomic gas lasers have earned a reputation for inefficiency because most of the best-known transitions, such as those in the helium-neon laser, are far above the ground state. That means only a small fraction of the excitation energy goes into the laser transition, and laser efficiency is low, making such gas lasers unsuitable for high-power operation. What makes alkali metals attractive is the pair of low-lying energy levels of their single valence electron (see Fig. 1). The laser scheme is simple—optically pump the electron from the ground state to the higher-energy 5 2P3/2 state by illuminating it on the D2 line, allow it to drop to the lower energy 5 2P1/2 state and stimulated emission will occur on the longer-wavelength D1 line as it drops back to the ground state.
The sodium D lines are well known for their strong yellow light and in principle could be used for a laser—if powerful yellow pump diodes were available. Krupke focused instead on potassium, cesium, and rubidium because their D lines are at longer wavelengths that can be pumped by near-infrared laser diodes (see table). Rubidium can be pumped on the 780 nm D2 line, readily available from aluminum-gallium-arsenide (AlGaAs) laser diodes, with laser emission on the 795-nm D1 line.The close spacing of the two levels makes the quantum defect-the difference between energy of the pump and emitted photons—remarkably small. For rubidium, the quantum defect is only 0.019, small compared to the 0.09 of ytterbium and the 0.24 of neodymium. Potassium has a much smaller quantum defect, 0.0044, but requires pumping at 766 nm, where diodes are not as powerful. Cesium has a quantum defect of 0.047, and a pump line at 856 nm. So far, most developers have concentrated on rubidium.
The peculiar dynamics of the quasi two-level system pose some challenges. Spontaneous emission rates from the upper levels are so high that alkali lasers don't store energy in the gain medium. Buffer gases such as the light hydrocarbon ethane are needed to drop electrons from the 5 2P3/2 level to the 5 2P1/2 level before spontaneous emission can occur. But experimental progress has been encouraging.
Experimental demonstrations
The first of the new alkali-vapor lasers was a rubidium laser that Krupke and colleagues pumped with a Ti:sapphire laser for experimental convenience in 2003. End-pumping a mixture of rubidium, helium, and ethane with 180 mW, they generated 30 mW at 795 nm, with overall optical conversion efficiency of 16% and slope power efficiency an impressive 54%. Demonstration of a cesium laser followed, with a 780 mW Ti:sapphire pump generating about 230 mW at 895 nm, for optical conversion efficiency of 35% and 59% slope efficiency.2
Diode pumping soon followed. End-pumping cesium with 400 mW from a narrow-line 852 nm diode generated 130 mW singlemode, for 32% optical conversion efficiency and 41% slope efficiency.3 End-pumping rubidium mixed with helium and ethane with 13 W from a 780 nm diode array generated about 1 W, with 10% slope efficiency.4
Boris Zhdanov and Randy Kinze at the US Air Force Academy (Colorado Springs, CO) were the first to reach 10 W CW output by pumping cesium with 25 W from an 852 nm diode array with linewidth of only 11 GHz, corresponding to 62% optical conversion and 68% slope efficiency.5 They also produced 17 W singlemode from rubidium by pumping it with 37 W from a pair of line-narrowed pump-diode arrays, reaching 46% optical conversion efficiency and 53% slope efficiency.6
Power levels have continued climbing. Last year, Jason Zweibeck of General Atomics Aeronautical Systems. (Livermore, CA) and Krupke reported that pumping rubidium with diode stacks generating 207 W of peak power at 14% duty cycle, and up to 145 W CW with 28% slope efficiency when pumping with 977 W from a diode array.7
Power scaling and laser design
The power levels and high conversion efficiencies are encouraging, but scaling diode-pumped alkali lasers to the kilowatt class and above poses plenty of challenges. At such power levels, issues such as optimizing energy transfer become increasingly important.One challenge is matching the relatively broad emission bands of laser diodes, typically in the 2- to 3-nm range, to the much narrower absorption lines of the alkali vapors. Early experiments achieved high efficiency by using narrow-line diodes, but that comes at a cost in lower diode efficiency. Another approach being explored is adding buffer gases such as helium to collisionally broaden the absorption spectrum to match the bandwidth of the pump diodes.
Another challenge is finding the best pump geometry. Most studies so far have used end pumping along the length of a waveguide cavity with a static fill of laser gas, which could achieve high conversion efficiency. However, this design depends on the thermal conductivity of the helium buffer gas to remove waste heat, so the width of the cavity must be limited to no more than a few millimeters in one dimension to avoid excessive thermal gradients that could degrade laser performance.Operating at higher power levels requires that the alkali vapor be flowed transversely through the pump volume, so waste heat can be removed outside of the laser cavity. Developers are working on two approaches. In one, end pumping of the laser medium and transverse flow is used—a design that limits the pump area but does not suffer from beam degradation if pumping is not uniform along the length of the cavity. The other approach uses transverse pumping, which increases the pump area but is vulnerable to beam degradation if pumping is not uniform (see Fig. 2).
The first demonstration of a flowing-gas alkali laser was by the US Air Force Research Laboratory (Kirtland AFB, NM), which in July 2010 reported "first light" from a flowing-gas diode-pumped alkali laser (see Fig. 3). Experiments with that system and others should lead to further power improvements.
Outlook
Times have changed since Krupke first envisioned diode-pumped alkali lasers for kilowatt-class industrial applications. Powerful fiber and thin-disk solid-state lasers have emerged that can dissipate heat well enough to generate high-quality kilowatt-class beams, while retaining other advantages of solid-state lasers. Bulk diode-pumped solid-state lasers have reached the 100 kW class for up to 5 min in military tests for potential weapons applications, although output from multiple apertures must be phaselocked to reach those powers, and thermal management remains challenging.
Krupke expects diode-pumped alkali lasers should scale to well over 100 kW with a single aperture delivering a high-quality beam, putting them in the running for tactical applications. They also became the leading candidate for megawatt-class weapons after the chemical oxygen iodine laser (COIL) used in the Airborne Laser failed to reach the range of a couple hundred kilometers needed to zap boost-phase ballistic missiles. Rather than trying to build a bigger and better COIL, the Missile Defense Agency is betting that diode-pumped alkali lasers will provide "a path forward to high efficiency, electrically-driven, compact, light-weight High Energy Lasers [to] address future threats." That will put serious money into the new technology, which may also bring dividends to the civilian community.
Big challenges remain, but after a long hiatus, alkali lasers have returned from technological limbo to offer exciting new possibilities. That adds an odd twist to the bizarre story of how military security officials refused in 1959 to give Gordon Gould a security clearance to work on a million-dollar project to try to build a laser based on his proposal. Alkali lasers seemed too impractical to bother classifying, so Gould was allowed to work on them. Somewhere Gould must be chuckling at the irony that alkali-vapor lasers have become the best shot at megawatt-class laser weapons.
REFERENCES
1. W.F. Krupke et al., "Resonance transition 795 nm rubidium laser," Opt. Lett., 28, 2336-2338 (2003).
2. R.J. Beach et al., "End-pumped continuouswave alkali vapor lasers: experiment, model, and power scaling," J. Opt. Soc. Am. B21, 12, 2151-2163 (2004).
3. T. Ehrenreich et al., "Diode pumped caesium laser," Electron. Lett., 41, 7, 47-48 (2005).
4. R.H. Page, R.J. Beach, and V.K. Kanz, "Multimode-diode-pumped gas (alkali-vapor) laser," Opt. Lett., 31, 3, 353-355 (2006).
5. B.V. Zhdanov and R.J. Knize, "Diode-pumped 10 W continuous wave cesium laser," Opt. Lett., 32, 15, 2167-2169 (2007).
6 B.V Zhdanov, A. Stooke, G. Boyadjian, A. Voci, and R.J. Knize, "Rubidium vapor laser pumped by two laser diode arrays," Opt. Lett., 33, 5, 414-415 (2008).
7. J. Zweiback, A. Komashko, and W.F. Krupke, "Alkali-vapor lasers," Proc. SPIE 7581, 75810G (2010); doi:10.1117/12.843594.
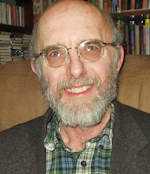
Jeff Hecht | Contributing Editor
Jeff Hecht is a regular contributing editor to Laser Focus World and has been covering the laser industry for 35 years. A prolific book author, Jeff's published works include “Understanding Fiber Optics,” “Understanding Lasers,” “The Laser Guidebook,” and “Beam Weapons: The Next Arms Race.” He also has written books on the histories of lasers and fiber optics, including “City of Light: The Story of Fiber Optics,” and “Beam: The Race to Make the Laser.” Find out more at jeffhecht.com.