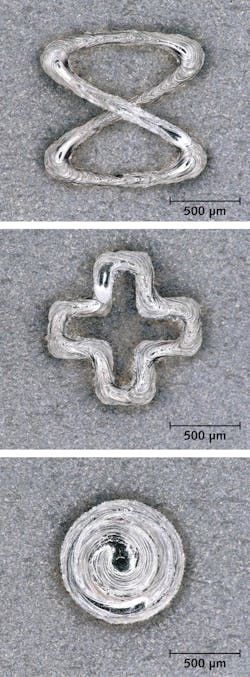
MICHAEL BERNDT, MARKUS DANNER, and ERNST TREFFERS
Laser welding of dissimilar metals, such as copper to aluminum or copper to stainless steel, has always presented challenges. However, the need for these types of welds is increasing, particularly in e-mobility and electronics applications. A typical example in this context is the laser welding of copper busbars to steel or aluminum battery components. This article presents work at Coherent (Santa Clara, CA) based on the company’s SmartWeld+ technology, which has demonstrated the ability to produce these types of laser welds at quality and throughput rates compatible with the requirements of automotive and electronics production.
Challenge of welding dissimilar materials
For the e-mobility example of busbar welding, there are several imperatives. First, the laser weld must demonstrate excellent electrical and heat conduction properties. Any compromise, particularly in electrical conductivity, reduces vehicle range, increases the required charging frequency, and raises the owner’s operating costs—all of these are undesirable outcomes. Additionally, busbar welds must possess high mechanical strength, as vehicular use subjects equipment to continuous vibration and occasional shock.
When laser welding identical or similar materials, a true metallurgical bond is formed at the weld seam. That is, the fusion zone has the same composition and, ideally, the same mechanical characteristics (for example, tensile strength, ductility, etc.) as the base material(s). In some cases, dissimilar materials do form stable alloys possessing desirable characteristics—for example, copper and zinc combine to make brass. However, many other materials either don’t combine to form metallurgically stable alloys, or don’t form alloys with acceptable properties.
If a true metallurgical bond cannot be formed between the metals in use, then one solution is to create a mechanical “entanglement”—that is, a spatial mixing of the dissimilar alloys so that they are physically held together in a manner that imparts sufficient mechanical strength to the connection. Think of this as creating a series of twisted “spikes” in the material that interpenetrate and hold the weld together.
One way of accomplishing this type of mechanical connection is by physically pressing the two parts together and then using a series of laser pulses to melt the two materials in a locally, spatially defined way. However, there’s really no control over melt pool dynamics using this approach and it results usually in an unsatisfactory surface quality. A good mechanical connection is simply achieved by making a sufficiently large number of these stitches. As a result, the process isn’t entirely consistent. Essentially, the same ends can be accomplished using ultrasonic welding rather than laser welding.
Traditional soldering is particularly disadvantageous for e-mobility use because the solder joints have a low melting point and exhibit poor mechanical strength and stability. Plus, solder often contains lead, which presents environmental concerns.
Laser beam control
Most fiber laser welding is done with larger, multimode, focused laser spots. This eliminates the problem of producing a weld seam that is too narrow, and which therefore may have insufficient mechanical strength. However, even a multimode laser spot still requires a relatively small physical gap between the parts, which necessitates better part edge preparation and fit-up by the manufacturer. These requirements, in turn, increase production costs.
Beam wobble—that is, rapid scanning of a small, focused, single- or multimode laser beam perpendicular to a weld seam (rather than a simple straight-line movement of the beam along the desired weld seam)—has already become established as a means for overcoming these limitations. Beam wobble increases the effective size of the beam, while still efficiently delivering the laser power intensity required for keyhole welding. Thus, beam wobble enables bridging of relatively large gap sizes, as well as more deterministic control of weld parameters, including seam width and penetration depth. The use of beam wobble with a single- or multimode spot has demonstrated superior results in several applications over traditional multimode spot welding.
Coherent’s particular embodiment of beam control, called SmartWeld+, comprises some technological advances over other versions of the method. In particular, it incorporates predictable energy input. It works with an infrared (1070 nm) focused single-mode beam with a spot size of 30 µm and expands the number and variety of oscillation patterns to include ellipses, spirals, and even more complex patterns (FIGURE 1). These patterns can be scaled in size, and automatically rotated to follow a weld path or contour. The energy distribution within a spot or seam is precisely controlled by adapting the scan speed along the trajectory within a micropattern, in some cases in combination with power modulation of the laser. This allows the individual adjustment of the energy input within different sections of a pattern or material and thus to define preheat, process, or cool-down areas and precisely manipulate weld pool dynamics. The result is an improvement in weld precision and repeatability, together with minimization of the heat-affected zone (HAZ) and low porosity. The examples shown here highlight the specific benefits of this technique.
Busbar and connector welding results
SIGMAclad is a multimaterial laminate specifically developed for use as an electrical connector in applications such as e-mobility. It was designed to replace nickel, which is commonly used for connecting lithium-ion batteries. While nickel does possess a favorable combination of mechanical, chemical, and electrical properties, its electrical resistance results in some heat generation, which therefore reduces efficiency. SIGMAclad is a five-layer sandwich of materials, specifically nickel, stainless steel, copper, stainless steel, and nickel.
Two different welding tests involving SIGMAclad were recently performed in a controlled study using SmartWeld+ (FIGURE 2). In the first, a 0.3-mm-thick SIGMAclad busbar was welded to another SIGMAclad strip of the same thickness without compromising the second copper layer. This represents the task of welding one busbar to an adjacent busbar. Next, the same SIGMAclad strip was welded to a 1-mm-thick nickel-plated copper sheet. This demonstrates the process of connecting a cylindrical battery to a busbar.For both welds, 250 to 350 W of average laser power was used, and the beam was scanned in a microspiral pattern. The total weld seam had a diameter of 1 mm, and a depth of 400 µm. Welding cycle time was 40 ms to produce a weld spot of 1 mm diameter.
The photos in FIGURE 2 show cross-sections of the two welds produced. In both cases, the welds possess a highly rectangular cross-section and, therefore, a strong and even bond. Furthermore, the weld stops at a well-defined depth, specifically at the second copper layer in the case of connecting the two busbars. This is a direct result of the ability to precisely control the energy input both spatially and temporally.
A second series of tests involved welding three sheets of 0.2-mm-thick nickel-plated copper to aluminum using 500 W of average laser power (FIGURE 3). The wobble pattern utilized in this case was an infinity or “figure 8” shape whose aspect ratio can be adjusted in relation to feed speed. The width of the weld seam was 1.4 mm over a length of 20 mm. It took 0.6 s to produce this weld, which had a depth of 1 mm.FIGURE 3 shows a top view and cross-section of this weld. Again, the weld shows excellent metallurgical homogeneity (low porosity) and very little taper in the top layer. No impact from the weld was seen on the back side of the aluminum sheet. Mechanical testing gave a shear strength of over 1700 N for this weld.
A third study (FIGURE 4) demonstrated the type of spot weld that battery manufacturers often need to produce an electrical connection to a cell. For this, a 0.2 mm copper strip was welded to 0.3-mm-thick stainless steel (typical of a cylindrical battery casing). Here, 500 W of average laser power was employed, and the beam was moved in a spiral pattern. The final spot weld had a 1 mm diameter, with a depth of about 300 µm. This weld took 26 ms to produce.FIGURE 4. This test involved laser spot welding a copper connector to a battery casing (a); the weld cross-section (b) shows that the materials were physically mixed, and the weld demonstrated adequate mechanical strength.
The photos in FIGURE 4 show that adequate mixing of the materials was obtained utilizing these process parameters. The weld demonstrated both high electrical conductivity and a shear strength of 150 N per weld spot.
Additionally, no thermal impact was observed on the inner side of the battery cell. This is due to careful control of energy input. This is of particular importance for batteries, as busbars are connected after they are filled with electrolyte. Heat input may result in damage of the lithium-ion cell and create an explosion hazard.
Conclusion
Accurate and highly dynamic beam control with SmartWeld+ has proven to be a significant advance in fiber laser welding technology, extending the utility of this economical and reliable laser source to a greater number of applications. Now, beam control has progressed past simple circular beam oscillations to encompass a wider range of patterns and also incorporate more sophisticated control of delivered power. Especially when combined with innovative assist gas delivery systems, this enables greater control over melt pool dynamics, producing welds of higher quality in a more deterministic fashion. All of this enables quality welding of dissimilar materials and thin sheets, as well as other challenging welding tasks in e-mobility and electronics production.
Dr. MICHAEL BERNDT ([email protected]), MARKUS DANNER, and ERNST TREFFERS are product line managers with industrial lasers and systems at Coherent, Munich, Germany; www.coherent.com.