Laser-based additively manufactured titanium-alloy parts are comparable in strength to forged pieces
Aerospace manufacturers, industries, and government agencies like the design freedom and convenience afforded from laser-based additive manufacturing. But do these parts have the same properties—especially strength—as those made using the more conventional subtractive method, being turned on a lathe from a solid stock? A recent study at the University of Illinois (U of I) at Urbana-Champaign conducted stress tests to find out.1
Additively manufactured cylinders were obtained made from Ti-6AL-4V, a titanium alloy which is about 90% titanium, 6% aluminum, and 4% vanadium, or Ti64 for short. Layers of the titanium alloy in the form of a powder are locally melted by a high-power laser and built up layer-by-layer into a shape guided by a CAD algorithm; each layer is 30 µm thick. As layers are added, they are melted by the laser and bonded to the ones below.
In the experiment, the cylinder is sandwiched between two steel bars that are long, strong, and relatively thick. Another bar, called a striker bar, is propelled using compressed air so that it hits the first bar. It transmits a stress pulse that then passes through the bar and into the sample, and the recorded signals graph a stress-strain curve for the Ti64 material, according to John Lambros, professor in the Department of Aerospace Engineering and director of the Advanced Materials Testing and Evaluation Laboratory at U of I.
Because of the additive method, there is a directionality to the microstructure of the cylinders, like a seam between each of the layers, creating a sort of grain structure to the material.
“Our original belief was that the layering would have a huge impact on the strength properties of the material,” Lambros says. “The structure is very different from traditionally forged material. The grains are more elongated and are bigger, reaching hundreds of microns. In the forged material, the grains are much smaller and more rounded. But the additive Ti64 didn’t break the way we thought it would. That was, for me, the most surprising thing. We found that they sometimes broke on the microstructural seams. These are planes that offer the least resistance. But we learned that the layer interface itself is pretty strong. The additive Ti64 actually breaks more or less how a regular material would break. What this means is that even under these extreme circumstances and loadings, it’s quite good. Current metal additive-manufacturing techniques produce much better parts than they did as recently as five years ago. Comparatively to earlier generations of builds, these newer additively manufactured metals are fantastic.”
Comparison to regular forged titanium
Lambros notes that the level of stress these materials were subjected to is not a level of compression it would be designed for, not for normal applications. They also tested conventional titanium using stock of regular forged titanium and did the same experiments. “The level of compressions we used in this experiment were similar to impact events, like those seen in a crash or explosion,” he says. “But for many applications, designers would want to have that information to fully understand the limits of the material.
“So far, what we have seen is that additively manufactured parts are good and reliable and consistent,” Lambros says. “And certainly as good, if not in some cases better, than regular material. The microstructure is very different -- the grains, for example. They look very different. There’s a lot of residual stress because these additively manufactured materials underwent a very violent past to get to this point—a torturous history similar to welding as lasers heat the layers as they are applied. They leave some stresses in there that would affect things. But again, apparently not that much in the end.”
Lambros adds that when parts are being certified for flight, they are examined from the beginning of their life -- meaning starting from how the original material was made down to the design of the part and how it’s used in the aircraft. A lot has to happen before a part obtains FAA approval.
“Although initially there were all sorts of problems with these AM [additive manufacturing] materials,” he says, “now it’s to the point that the product is actually quite good. It’s comparable to what you would get through your typically forged or machined material and in some instances, it’s perhaps even better, bringing additively manufactured parts one step closer to certification.”
This work was supported by a grant from the University of Illinois Research Board. The test cylinders were made by the Illinois Applied Research Institute, an initiative of The Grainger College of Engineering at the University of Illinois at Urbana-Champaign.
Source: https://www.alphagalileo.org/en-gb/Item-Display/ItemId/184553?returnurl=https://www.alphagalileo.org/en-gb/Item-Display/ItemId/184553
REFERENCE
1. Waymel, R.F., Chew, H.B., and Lambros, J., Exp Mech (2019) 59: 829. https://doi.org/10.1007/s11340-019-00506-2
Got optics- and photonics-related news to share with us? Contact John Wallace, Senior Editor, Laser Focus World
Get more like this delivered right to your inbox
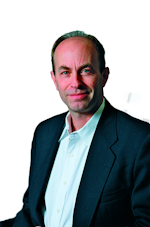
John Wallace | Senior Technical Editor (1998-2022)
John Wallace was with Laser Focus World for nearly 25 years, retiring in late June 2022. He obtained a bachelor's degree in mechanical engineering and physics at Rutgers University and a master's in optical engineering at the University of Rochester. Before becoming an editor, John worked as an engineer at RCA, Exxon, Eastman Kodak, and GCA Corporation.