The use of laser diodes is leading to handheld medical instruments
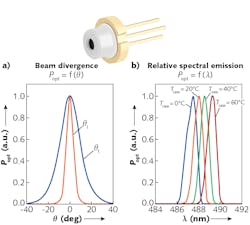
Advances in laser diodes are revolutionizing the biomedical field by bringing diagnostic instruments found only in medical laboratories directly to consumers. Laser diodes offer many benefits over other light sources by enabling biological instruments to be smaller, less complex, and more cost-effective than ever before.
The output power of a multimode laser diode ranges from milliwatt to multiwatt levels. The strong competition to increase the output power of diodes and bars has led to a strong growth trend. While increases in diode bar power have led to new applications in materials processing, some emerging applications demand the enhancement of other laser-diode parameters like wavelength stability, beam quality, and polarization.
The focus is now on miniaturization, efficiency, and single-mode beams. It’s possible to use optics (although not without tradeoffs) to clean multimode laser-diode beams into a true single mode. Many are not available in ideal wavelengths, which prevents their mass adoption as alternatives for gas ion lasers and various visible-line applications. Advances in transverse single-mode visible and infrared laser diodes with efficiencies up to 30%,1 high wavelength stability, polarization, and long coherence length make many of these workarounds unnecessary (see Fig. 1).
Beam profile and beam quality for imaging: What are the challenges?
Infrared lasers work well for deep penetration such as hair removal or nanoparticle-assisted cancer treatment. Visible-laser wavelengths are easily absorbed by blood, and therefore work well for tattoo removal and pulse oximetry. These are more power-driven applications and less optically demanding. When using lasers for imaging, gas ion lasers in flow cytometry typically operate at wavelengths of 350 to 361, 457, 488, 514, 543, 568, and 638 nm. Red and green helium-neon (HeNe) lasers and dye lasers have widely been used for biological laser imaging, producing a high-quality, radially symmetric TEM00 (Gaussian) mode. Laser diodes, however, were typically associated with an elliptical and very noisy profile having various undesirable nodes and multiple modes observed, requiring some aspect ratio correction and beam cleanup.
To do fluorescence or biological imaging measurements with adequate resolution and signal-to-noise ratios, an adequate-power Gaussian beam should be focused to a diffraction-limited spot. For example, the Rayleigh criterion for a diffraction-limited vision of an iris is:
ƟR=1.22 λ/d [rad] (1)
where λ is wavelength and d is the iris diameter.
With appropriate optics, the beam is nearly collimated when the waist of the beam is near the exit of the laser packaging. Packaged diode lasers commonly have beam diameters of 0.5 to 2 mm and a beam divergence of less than 1 mrad. Unfortunately, diode lasers may require much-smaller beam diameters that cannot satisfy the requirements of confocal microscopy. If Wo is the beam radius at the waist and λ is the laser wavelength, the divergence half angle is:
ƟR = λ/(πWo) (2)
The depth of focus is the distance between the points in which the beam area is twice the area at the waist. The depth of focus or confocal parameter is defined as 2zo, where zo is the Rayleigh range and is equal to √2 Wo (see Fig. 2). What is then seen is that the depth of focus is no longer useful when trying to focus down a diverging or multimodal diode laser to a spot size of far less than 500 μm.The ideal requirement is a true Gaussian beam with an equal divergence from theoretical in both the y and x axes. This property, M2 = (ƟRπWo)/λ for laser diodes,2 is coupled with an idealized diffraction-limited lens exhibiting no aberrations. A uniform illumination profile with an M2 = 1 is then perfectly Gaussian and radially symmetric. A typical elliptical laser-diode beam nowadays has an M2 of about 1.2. However, even when cropping the fast axis to the same divergence as the slow axis, such a beam historically might not serve as an ideal illumination source. The beam profile could exhibit noise and modes emerging, resulting in a distributed light intensity that was not uniform and noise-free.
Some applications benefit from the highly polarized and coherent light of laser diodes, but not all. Coherence-induced speckle can cause serious imaging issues. Single-mode laser diodes now exhibit >99% polarized light and a 1–2 nm bandwidth at full-width half-maximum (FWHM). If desired, these two inherent properties can now also be altered with thoughtful multiple laser arrangements in packaging.3 Speckle can also be mitigated using novel multi-emitter lasers or wavelength-offset combinations.
Another alternative approach when using a multimodal laser diode is rastering across the field of view with a tightly focused beam in what is known as scanning laser cytometers. These types of systems produce moderate-quality images. The concentrated beam allows for the uniform illumination required to image through a layer of cells, but is not very high resolution with the typical 2–5 μm spot size (see Fig. 3) and exhibits an extremely short working distance. It is also common in laser transmission spectroscopy and flow cytometry to enhance the ability to detect target species using DNA, which has been attached onto plastic micro- and nanoparticles and requires a spot size of 1 μm or less. Once again, it is seen that a highly focused laser is needed to characterize the small beads or cells, so the solutions created just as many problems as they solve.Wavelengths, power, and filters for good SNR
As seen in Table 1, there is a wide variety of excitation wavelengths used to pump fluorochromes.4 The list becomes longer when also considering fluorophores. Biologists have made do with the excitation lines historically available in gas ion and dye lasers. Osram created the direct-transition 488 nm laser diode to provide a solid-state version of the highly inefficient and very large gas ion laser, which is leading to the adoption of handheld biological instrumentation. As the number of applications grows for medical applications (see Table 2), the laser diode’s available wavelengths expand as well.Biological instrumentation finds its limitations not only on the illumination side, but also on the filtering side as well. High-pass filters with a sharp cutoff are expensive and custom filters can be very cost-prohibitive. Instrumentation designers now find themselves having to sacrifice signal-to-noise ratio (SNR) because the excitation wavelength does not provide the adequate optical energy or wavelength stability to reliably probe samples. One key advance in single-mode laser diodes is an increase in power, which provides better SNR. With an efficiency of up to 30%, laser diodes once again provide appealing benefits compared to the 0.1% efficiency of a gas ion laser.
Single-mode laser diodes are maturing, filling an unmet need in biological imaging. The high power and efficiency of direct-transition laser diodes, which are a fraction of the size and cost of a gas ion laser, make portable options a reality. The beam quality achieved is adequate for laser imaging without sacrificing depth of focus. As finely tuned excitation lines become available, a revolution in flow cytometry and laser transmission spectroscopy can be expected.
REFERENCES
1. H. König et al., Proc. SPIE, 10939, 109390C (2019).
2. See https://bit.ly/OsramRef2.
3. A. Russell et al., “Laser packaging architecture which overcomes challenges in AR imaging,” SPIE Photonics West 2020 presentation, San Francisco, CA (Feb. 2020).
4. See https://unc.live/2AeEgN1.
Thomas Brandes | Senior Marketing Manager, Osram Opto Semiconductors
Thomas Brandes is Senior Marketing Manager at Osram Opto Semiconductors (Novi, MI).
Ann Russell | Senior Applications Engineer, Osram Opto Semiconductors North America
Ann Russell is senior applications engineer at Osram Opto Semiconductors North America (Sunnyvale, CA).