Picosecond and femtosecond fiber lasers serve industry and science
The advent of picosecond and femtosecond fiber lasers has provided compact and easy-to-maintain ultrafast tools for industry and academia. Applications of these lasers range from materials processing to microscopy and other scientific uses. Placing these lasers at the technological leading-edge are advances that include polarization-maintaining and photonic-crystal fibers, as well as cavity and extra-cavity elements such as passive and active mode-locking, semiconductor saturable absorber mirrors, and frequency conversion. This article discusses ultrafast fiber lasers and presents numerous product examples and applications.
Spectral coverage from 500 to 15,000 nm
Every ultrafast fiber laser manufacturer adds its own spin to the technology. For example, Menlo Systems (Planegg, Germany) has developed its own mode-locking approach (called “Figure 9,” which it has patented), based on an all polarization-maintaining-fiber design with no lifetime-limiting components. Christian Mauser, product manager for femtosecond fiber lasers at Menlo Systems, explains that the additive-pulse mode-locking technology is based on a nonlinear amplified loop mirror, which acts as a very fast artificial saturable absorber,1, 2 and which he says has several advantages compared to other techniques like semiconductor saturable absorber mirrors (SESAMs).3
“Due to the optimized design, lowest timing jitter and phase noise, combined with very low relative intensity noise (RIN), can be achieved with a simple, robust, and reliable operation at the same time,” says Mauser. “The fiber layout enables a very compact footprint with temperature- and vibration-insensitive operation, which leads to maintenance-free operation. Due to the ultrashort femtosecond pulses with very high peak powers, a huge spectral coverage from 500 up to 15,000 nm can be achieved via various nonlinear processes such as higher harmonic, supercontinuum, or difference-frequency generation. Femtosecond laser oscillators with starting wavelengths from 930, 1040, 1560, and 2050 nm can be realized. With subsequent amplification and pulse compression, high peak powers can be achieved with several watts of average output power and pulse widths of 40 to 400 fs. Using additional nonlinear processes, the wavelength and the pulse width can be modified.”
The repetition rate and the carrier-envelope frequency can be adjusted with intracavity actuators in a wide range, enabling a so-called (fully) stabilized optical frequency comb, notes Mauser. This is particularly important for applications in spectroscopy and metrology with stabilized lasers, such as the interrogation of optical transitions in atomic clocks. Optimization of optics and electronics leads to timing jitter at the attosecond level and very low phase noise.
In the past decades, femtosecond titanium sapphire (Ti:sapphire)-based laser systems were the dominating light source for multiphoton imaging applications, but they are known for their complexity and demands in lab space and infrastructure. Fiber lasers serve as a capable replacement here and are particularly beneficial for new microscopy applications such as portable nonlinear endoscopy systems capable of imaging neuronal activity in live animals in an unprecedented flexible way.4 Fiber-based laser systems also present new opportunities to improve signal-generation efficiency in multiphoton imaging; for example, the pulse repetition frequency can be chosen over a large range. Reducing the repetition frequency allows an increase of the peak power and thus the generated nonlinear signal, while keeping the average power and thereby the thermal damage on the sample low.
Moreover, longer wavelengths with a multiphoton absorption mechanism provide high image contrast in deeper regions of the sample even at very low excitation powers, notes Mauser. Especially for two-photon microscopy on thick samples, longer wavelengths can be highly beneficial to reduce scattering and improve contrast ratio. Figure 1 shows an example of a multiphoton excitation fluorescence image of a 300 µm thick mouse-brain sample. The sample is stained with Alexa 647 and is excited with laser pulses from a Ti:sapphire system at 780 nm and a wavelength-shifted ytterbium-doped fiber laser (Menlo systems YLMO) at 1300 nm. The advantage of the longer wavelength excitation is clearly visible: High image contrast also in deeper regions of the sample, even at very low excitation powers.
High-speed cutting
Spectra-Physics (MKS Instruments; Santa Clara, CA) develops and manufactures high-power picosecond and femtosecond lasers for industrial materials processing, covering infrared (IR), green, and ultraviolet (UV) wavelengths. “Our hybrid-fiber laser designs feature high pulse energies (for example, greater than 600 µJ for IR femtosecond pulses), wide operating ranges (single shot to greater than 10 MHz), and ‘TimeShift’ capability for flexible pulse programmability,” says Scott White, director of product marketing. “These capabilities enable high-speed micromachining of a wide range of today’s complex materials, such as OLED displays, brittle materials, thin films, flexible printed circuit boards (FPCBs), and more.”
The company’s 50 W UV picosecond fiber laser, the IceFyre 355-50, produces 40 μJ/pulse at 1.25 MHz with typical pulse widths of 10 ps, and can operate from single shot to 10 MHz. With a hybrid fiber design, the laser’s TimeShift picosecond programmable burst-mode technology allows users to create their own waveforms to optimize process speed and quality, and can produce pulse energies of hundreds of microjoules in burst mode. The laser has pulse-on-demand (POD) and position-synchronized output (PSO) triggering with “the lowest timing jitter in its class for high-quality processing at ultrahigh scan speeds (for example, when using a polygon scanner),” says White, adding that the laser is capable of processing a wide range of materials such as glass, sapphire, FPCB, and flat-panel display materials.
The IceFyre 355-50 can be used for high-speed cutting of flexible display material, such as OLED, polyethylene terephthalate (PET), and cyclo-olefin polymer (COP) used in smartphones. OLED manufacturing typically involves processing a layered stack of PET/COP with adhesives between the layers. The finished stack must be cut and contoured to exact requirements. Picosecond UV lasers have been used to obtain the quality required in flexible OLED cutting for several years, but the challenge has been to cut the material at high speeds to meet production demands for these consumer electronics. “Because of its higher power at higher rep rates, the IceFyre 355-50 can cut flexible OLED material with the same pulse energy and pulse width as previous and more costly UV picosecond lasers, but at speeds almost 90% faster,” notes White.
Tunable pulse width optimizes scribing
Well known for its photonic-crystal fiber (PCF) technology, NKT Photonics (Birkerød, Denmark) has applied its knowledge to its fiber lasers—for example, in the form of the aeroPULSE ultrafast fiber laser platform, which is based on the company’s PCF amplifier technology. As described by Carsten Thomsen, the company’s senior VP of ultrafast lasers, standard picosecond models are offered with output powers of 10 W (aeroPULSE PS10) and 40 W (aeroPULSE PS40) at 1030 nm and 5 ps pulse width (customizable between 5 and 50 ps), with narrow-bandwidth versions available for applications where, for example, efficient external frequency conversion is required.
The aeroPULSE FS series builds on the picosecond platform by harnessing the company’s Optocage technology to provide femtosecond ultrafast-laser output at pulse durations shorter than 500 fs, with high pointing stability and output powers of 20 to 50 W. Green and UV frequency-conversion modules are available for the aeroPULSE platform, with single or multiwavelength options.
The latest aeroPULSE FS-50 femtosecond fiber laser provides 50 W of output power at 1030 nm, with 40 µJ of pulse energy, says Thomsen. Additional features to optimize laser-processing conditions include burst mode, power attenuation, repetition-rate selection (single shot to 50 MHz), pulse-width selection (<500 fs to 3 ps), and gate and event trigger control. Green (515 nm) and UV (343 nm) harmonics modules allow extra flexibility for processing thermally sensitive or brittle materials. “The laser is ideal for applications targeting thin-film cutting, glass marking and cutting, medical stent manufacture, IC package and PCB cutting, scribing of low-k materials, OPA pumping for bioimaging, surface-treatment medication, and corrosive-resistant marking,” Thomsen explains.For applications in the display industry, low-k, temperature-sensitive materials require ultrafast processes to ensure that thermal processes are kept to a minimum, especially as next-generation OLED and flexible displays comprise of multilayer stacks of thin (less than 200 µm) dielectric materials. The ability to tune the aeroPULSE FS-50 laser’s pulse width provides flexibility for the user to optimize the scribing process, especially when working with material stacks of differing materials. The added flexibility of also being able to tune the repetition rate and pulse energy, and the possibility of using burst mode, allows the customer to refine the scribing/cutting process and increase throughput. With the availability of green and UV as options, smaller spot sizes with further reduction in thermal loads increase the precision of delivering the pulse energy and also increase the yield and reliability of the processed material due to the reduction of microcracks and uneven processed kerfs.
Microscale printing
Calmar Laser (Palo Alto, CA) has knowledge and a strong patent portfolio in a wide range of technologies, including passively mode-locked femtosecond fiber lasers, actively mode-locked picosecond fiber lasers, chirped pulse amplifiers, nonlinear wavelength conversion, large-core and double-clad erbium/ytterbium fiber amplifiers, optical parametric amplifiers, and laser electronics hardware and software, notes Tony Lin, the company’s founder and president. Ultrafast fiber laser and fiber amplifier systems made by Calmar Laser are targeted for OEM, B2B, and research customers in the biomedical, semiconductor/microelectronics, telecommunications, industrial, and scientific research market segments.
The company has three major product platforms: first, OEM modules, which are robust, highly customized fiber lasers for incorporation as seed sources/amplifier modules into other manufacturer’s higher power laser platforms; second, benchtop systems designed for test and measurement applications in manufacturing or R&D environments; and third, high-power systems developed for specific applications across different market sectors.
A new series of femtosecond sources with gigahertz low-jitter synchronization signals has recently been added to the company’s low-power Mendocino benchtop platform. With wavelength options of 780, 850, 1310, and 1550 nm, pulse widths of less than 0.3 ps, and timing jitter as low as 200 fs, this new product line offering is well suited to be an optical source for high-speed transceiver conformance testing and photodiode characterization.
The Carmel X-780 is the smallest high-power femtosecond fiber laser on the market with up to 1 W of output power at 780 nm and pulse widths of less than 90 fs. The building block of the Carmel X-series is an ultrafast Er:fiber with a Calmar-proprietary saturable-absorber technology to deliver reproducible and reliable mode-locking at turn-on. An all fiber-based chirped-pulse amplification scheme is used to provide higher pulse energies/higher average power. Chirped 1.55 μm pulses are delivered through an armored fiber cable to a palm-sized laser head, where they are compressed and converted to a 780 nm output useful for bioimaging, two-photon microscopy, optical metrology, 3D nanoprinting, terahertz imaging, ophthalmology, and so on.
UpNano (Vienna, Austria) is a pioneer in the development of novel ultrafast high-resolution 3D printing systems. In 2019, the company introduced the first desktop 3D nano- and microprinting platform, NanoOne, for manufacturing prototypes and low-volume production runs of microcomponents (see Fig. 2). One of the most critical components in designing such a system was the ultrafast laser source. “In order to achieve up to 100 times higher throughput with high scanning velocities, we required an ultra-stable ultrafast laser source with over 1 W of output power,” says Peter Gruber, cofounder and head of technology at UpNano. “In addition, we needed a footprint that would support our compact desktop design. After a careful evaluation, we concluded that the Carmel X-780 was only commercial laser source that offered the required power, stable femtosecond output pulses, and an all air-cooled, compact form factor. It is a key enabler for our platform.”Ultrafast fiber lasers for science
The wide variety of scientific lasers produced by TOPTICA Photonics (Munich, Germany) has ensured their use in labs worldwide, which goes as well for the company’s ultrafast fiber lasers. “These systems gain their stability by relying on SESAM locking in erbium oscillators,” says Adam Heiniger, ultrafast applications scientist at TOPTICA. “We then amplify in erbium to get high power at 1560 nm, which allows us to use frequency-conversion tricks to generate watt-level powers at 780, 920, and 1050 nm. These are important wavelengths for multiphoton imaging. We can also build multiple-output, synchronized multicolor systems that are seeded from a single oscillator for pump-probe spectroscopy or coherent Raman chemical imaging, and these fiber-based systems are much more stable and less costly than similar systems that rely on solid-state lasers.”Because pump-probe setups sometimes suffer from slow mechanical delay lines, TOPTICA can implement asynchronous optical sampling (ASOPS), where the repetition rate offset between two oscillators is carefully controlled to rapidly change the time delay between pump and probe pulses. In addition, Heiniger notes that the company has mastered arbitrary pulse picking of microjoule-class fiber lasers down to the single-pulse level, which is vital for raster-scanned laser material processing. Usually raster-scan systems cannot maintain a fixed velocity as the scan turns corners, and the variable repetition rate of TOPTICA’s ultrafast fiber lasers can compensate to maintain a fixed number of pulses per unit distance even as the scan slows.
“Two-photon fluorescence microscopy (see Fig. 3) is used to monitor live brain activity, and the best fluorescent probes used for this purpose, GFP or GCaMP, have optimal two-photon excitation around 920 nm,” says Heiniger. “Until now, only Ti:sapphire lasers have been capable of providing the necessary pulse durations and peak and average powers for this application. TOPTICA’s FemtoFiber ultra 920 is the only fiber laser that can match the performance of a Ti:sapphire laser, and as a fiber laser it is much more cost-efficient, requires no regular maintenance and no water cooling, and requires only a fraction of the space on the optical table.”
It is challenging to provide this wavelength with high power and short pulse duration using fiber, explains Heiniger. “Our exact approach is proprietary, but to reach 920 nm from a 1560 nm oscillator we had to strongly expand our frequency conversion toolbox,” he says. “Special care was taken to design the necessary spatial beam parameters and a short, ‘clean’ pulse shape: In our laser, typically 99% of the pulse energy is temporally compressed in the main pulse, which results in very effective usage of every photon for two-photon fluorescence excitation rather than unwanted thermal load on the live brain tissue. Our ultra 920 can provide 1.5 W average power at 80 MHz repetition rate, with less than 100 fs pulse duration and greater than 188 kW peak power. An important figure of merit that measures pulse shape and its impact on two-photon imaging and live cell heating is the ratio of peak to average power, which is more than 125 kW/W for our system.”
Weijian Zong and his colleagues at the Moser Lab (Trondheim, Norway) use the FemtoFiber ultra 920 in their research into neuron activity in the brain of a free-moving mouse. For this application, the laser is coupled into a special glass fiber that guides the beam into a small microscope, which is directly attached to the head of the free-moving mouse. “The ‘Clean-Pulse’ technology of the laser in combination with integrated dispersion precompensation is key for the approach providing insights into the natural brain function of the animal,” notes Heiniger. “In addition, the intrinsically quiet fiber laser design strongly reduces unwanted background noise to minimize the disturbance to the animal by the laser.”
FCPA pioneer
IMRA America (Ann Arbor, MI), which is the company that pioneered fiber chirped-pulse-amplified (FCPA) technology and introduced it into commercial applications for materials processing, manufactures FCPA mode-locked femtosecond laser sources, as noted by Emily Grish, marketing and sales specialist at IMRA. “The laser parameters of the DE and DX Series products are optimized for unique femtosecond applications,” she says. “The output wavelengths are near 1040 nm and optionally run at 520 and 347 nm, providing femtosecond and shorter wavelength combinations. The lasers feature variable repetition rates, pulse bursting, and pulse-picking options.”
The DE 2050 ULTRA model is engineered based on one of the all-fiber based FCPA designs at IMRA, integrating green and UV wavelengths all in one system. A femtosecond pulse energy of up to 50 µJ and an average power up to 20 W in IR forms the baseline laser parameters, which can then be converted to second and third harmonics. Targeted uses are broad applications from precision marking and surface trimming to welding of transparent parts.
The DE 2050 ULTRA is ideal for welding of glass or other transparent materials, says Grish. Welding is achieved by melting the material precisely at the interface where the pieces meet without affecting the surrounding area. Since the process occurs at room temperature and without additional material or chemicals, the ULTRA enables in-line processes without changing the manufacturing flow to integrate other delicate materials. An example is areal seam seals of glass plates incorporating sensoric devices.
Next-generation SESAM technology
All ultrafast fiber lasers made by EKSPLA (Vilnius, Lithuania) use oscillators with SESAMs for passive mode locking and polarization-maintaining ytterbium-doped fibers. “SESAM-based fiber oscillators have number of advantages, such as easy self-starting, high repetition rates, and high stability; however, a major drawback of this technology is a comparatively low lifetime caused by a degradation of a SESAM,” says Aldas Juronis, head of the OEM lasers program at EKSPLA. “To overcome this drawback, our engineers have developed and tested a unique recipe for growing semiconductor wafers for SESAM devices with extremely long lifetimes. These new-generation SESAMs have already been tested for more than 15,000 hours of nonstop operation without any signs of early degradation. Based on this new SESAM technology, we already have started redesigning our entire family of fiber lasers to enable their 24/7/365 uninterrupted operation.”
The company has three fiber-laser product groups: the LightWire FPS series, which are 1064 nm picosecond lasers with average optical powers ranging from 1.5 to 250 mW (the most-powerful model FPS200 can be equipped with a 532 nm second-harmonic option); the LightWire FFS series is 1030 nm chirped picosecond (for seeding CPA systems) as well as 1064 nm lasers with average optical powers ranging from 50 to 200 mW and pulse durations shorter than 130 fs; and the FemtoLux 3 laser with tunable pulse duration in a range of less than 300 fs to 5 ps and a maximum optical power of more than 3 W at 1030 nm. This laser can be equipped with the second-harmonic option.
“One of the first fiber lasers that will be upgraded to the new extremely-long-lifetime oscillator will be the FemtoLux 3 laser,” says Juronis. “This is an industrial-grade, compact, passively air-cooled laser with a maximum pulse energy of more than 3 µJ (more than 10 µJ in burst mode), excellent beam spatial parameters, a tunable repetition rate from 10 kHz to 5 MHz, integrated pulse picker, and output power attenuator. This laser was proven by a number of our customers as a versatile tool both for scientific applications such as second-harmonic microscopy and femtosecond OPO/OPA pumping, and for industrial use for multiphoton photopolymerization or intravolume marking of transparent materials.”
Researchers at the Institute of Physics at Jagiellonian University (Krakow, Poland) have tested direct laser writing with the FemtoLux 3 laser in various glass matrices containing implemented silver ions (see Fig. 4). Such silver-containing glasses are promising candidates for many photonic applications due to the unique optical properties of silver nanoclusters and/or silver metallic nanoparticles.Direct output in the mid-IR
Ultrafast fiber-laser systems typically emit in the near-IR or visible spectral regions, but Femtum (Québec City, QC, Canada) is producing the first generation of fiber lasers and fiber amplifiers that emit directly in the mid-IR (2800 nm and above). The company’s products include the Ultra 2800, a 2800 nm femtosecond fiber laser; the Amp 2800, a 2800 nm fiber amplifier with gain up to 25 dB in one-stage configuration and up to 40 dB in two-stage configuration; and the UltraTune 3400, a watt-level femtosecond fiber laser tunable from 2.8 to 3.5 µm with pulse durations down to 150 fs and energies and peak powers up to 30 nJ and 100 kW, respectively. “Higher power and high pulse energy (micro- and millijoule) laser systems are coming soon,” says Simon Duval, cofounder and CTO of Femtum.
Due to its high beam quality and fiberized output, the Ultratune 3400 is a simple ultrafast laser that can be tuned precisely for optimal interaction with organic materials like polymers or biological tissues, notes Duval; uses include selective ablation of thin films and precise surface modification of polymers. The laser can also be tuned to the fundamental resonances of many molecules, including water and methane for frequency-comb applications, mid-IR microscopy, hyperspectral imaging, and other spectroscopy-related applications.
The Ultratune 3400 has been precisely tuned to the absorption peaks of two important industrial polymers (PMMA and PET) to generate on-surface waveguides inside such materials. Surface waveguides in these biocompatible materials can lead to the effective manufacturing of low-cost photonic devices and sensors for substance identification, as well as temperature and pressure monitoring. At higher powers, smooth and precise micrometer-scale surface modifications have also been observed, says Duval. Coupled with a galvo scanner head or motorized stages, arbitrary patterns can be quickly and precisely printed.
The Ultratune 3400 is also used as a scientific tool for experiments in nonlinear optics, including supercontinuum generation in chalcogenide fibers. A coherent quasi-watt-level mid-IR supercontinuum source extending from 2.5 to 5 µm was generated in such a fiber due to the long wavelength of this mode-locked laser. The highly stable supercontinuum source can be of great interest for mid-IR spectral-domain optical coherence tomography (OCT) or frequency-comb applications.
REFERENCES
1. W. Hänsel et al., Appl. Phys. B, 123, 41 (2017); doi:10.1007/s00340-016-6598-2.
2. J. Kim et al., Adv. Opt. Photonics (2016); doi:10.1364/aop.8.000465.
3. N. Raabe et al., Opt. Lett., 42, 6, 1068 (2017); doi:10.1364/ol.42.001068.
4. A. Lombardini et al., Light Sci. Appl., 7, 10 (2018).
For More Information
Companies mentioned in this article include:
Calmar Laser
Palo Alto, CA
EKSPLA
Vilnius, Lithuania
Femtum
Québec City, QC, Canada
IMRA America
Ann Arbor, MI
Menlo Systems
Planegg, Germany
NKT Photonics
Birkerød, Denmark
Spectra-Physics (MKS Instruments)
Santa Clara, CA
TOPTICA Photonics
Munich, Germany
UpNano
Vienna, Austria
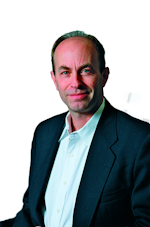
John Wallace | Senior Technical Editor (1998-2022)
John Wallace was with Laser Focus World for nearly 25 years, retiring in late June 2022. He obtained a bachelor's degree in mechanical engineering and physics at Rutgers University and a master's in optical engineering at the University of Rochester. Before becoming an editor, John worked as an engineer at RCA, Exxon, Eastman Kodak, and GCA Corporation.