It has long been known that laser light can be used to cool some solid-state substances via anti-Stokes fluorescence, which is a type of fluorescence that has a wavelength shorter than that of the exciting wavelength, thus carrying energy away. This phenomenon has allowed researchers to cool solids of macroscopic size by tens of degrees Kelvin. It has also allowed researchers to speculate on a so-called “athermal” laser, or one that cools itself via anti-Stokes fluorescence such that all potential heat energy produced by laser inefficiencies is carried off.
This achievement would be especially noteworthy if it were to occur in a silica-based laser—in other words, the type of gain material used in virtually all fiber lasers. Such a laser could be used, for example, to create advanced fiber amplifiers for datacom and telecom applications. Currently, this amplification process generates heat that degrades the quality of the light signal; using a self-cooled fiber would eliminate this problem.
But finding the right silica composition has proven elusive to the point where some experts thought achieving this goal was highly unlikely, if not impossible. Now, though, a team of researchers from Stanford University (Palo Alto, CA), Mid Sweden University (Sundsvall, Sweden), the University of Illinois at Urbana-Champaign (Urbana, IL), Clemson University (Clemson, SC), and Université Laval (Québec City, QC, Canada) has found the right composition: fused silica doped with 2.06 wt.% of ytterbium (Yb3+) and co-doped with aluminum oxide (Al2O3) and fluorine (F−).1, 2
The fiber used in their experiment had a 21 μm core, a numerical aperture of 0.07, a radiative lifetime of 890 μs, a nonradiative lifetime of about three years, a quenching lifetime of about 34 ms, and a low absorptive loss of 10 dB/km (to reduce the amount of heat produced that needed to be carried away). The fiber was pumped at one of 12 wavelengths between 1020 and 1070 nm; the fiber itself had a mean fluorescence wavelength of 1002.5 nm, providing the wavelength difference needed to carry energy away.
Experimental confirmation came when Stanford graduate student Jennifer Knall, up late at night, lowered the energy of the light used in the experiment by increasing the wavelength of the pump from 1020 nm to 1040 nm; the result was the first observed temperature dip due to self-cooling in silica (see figure).
Since their first breakthrough, the researchers have found two more silica fiber compositions that self-cool, and Knall has used the best-performing candidate to create a cooled fiber amplifier. She has been able to amplify laser light more than 40-fold while maintaining a negative average temperature change along the length of the fiber. Whereas the cooling tests proved that laser cooling in silica is possible, this fiber amplifier shows that it is also undeniably useful in practice, as the temperature of a self-cooling silica fiber laser doesn’t fluctuate, so the frequency and power of the light that they emit are more stable over time than lasers with external cooling, resulting in a more consistent output wavelength.
Right now, the researchers extract around 4% of the energy they inject into the fibers. This makes it unlikely that the fibers would be adopted for high-power applications without first increasing this low efficiency, but the researchers see many opportunities for extremely stable lasers in lower-power applications such as extremely precise metrology.
“How far we can take this technology will depend on how much researchers can push the materials science,” says Michel Digonnet, one of the Stanford researchers. “This is just the tip of the iceberg.”
REFERENCES
1. J. Knall et al., Opt. Lett. (2020); https://doi.org/10.1364/ol.384658.
2. J. Knall et al., Opt. Lett. (2020); https://doi.org/10.1364/ol.395513.
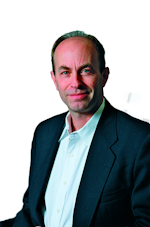
John Wallace | Senior Technical Editor (1998-2022)
John Wallace was with Laser Focus World for nearly 25 years, retiring in late June 2022. He obtained a bachelor's degree in mechanical engineering and physics at Rutgers University and a master's in optical engineering at the University of Rochester. Before becoming an editor, John worked as an engineer at RCA, Exxon, Eastman Kodak, and GCA Corporation.