Gas Lasers: CO2 lasers - progressing from a varied past to an application-specific future
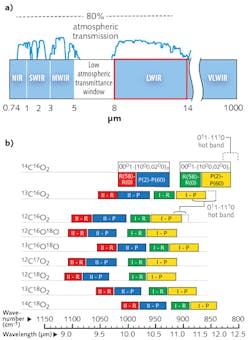
YONG ZHANG and TIM KILLEEN
Invented by C. Kumar N. Patel in 1964 at Bell Laboratories, carbon-dioxide (CO2) lasers are an ancient technology by photonics industry standards. But despite a long history, CO2 laser technology has survived and thrived because of its unique combination of wavelength, power, and spectral purity.
Because many natural and synthetic materials have strong characteristic absorptions in the 9–12 μm spectral range spanned by CO2 lasers, there are numerous opportunities in materials processing and spectral analysis. These wavelengths are also contained in an important window for atmospheric transmission, ideal for many sensing and ranging applications (see Fig. 1).1
A typical CO2 laser consists of a volume of electric discharge with a gas mixture that includes CO2 molecules. Because the energy levels of molecular vibration and rotation are close together, photons emitted as a result of transitions between these levels are low in energy and long in wavelength compared to visible and near-infrared (NIR) light.
Carbon-dioxide lasers can provide power levels from milliwatts to tens of kilowatts, making them equally suited to instrumentation or brute-force cutting. And because CO2 lasers have very high spectral purity, with <1 kHz of radiated linewidth without a power tradeoff, conversion efficiencies of 10% are possible. These traits allow CO2 lasers to tackle emerging applications in materials processing, light detection and ranging (lidar), thermal vision assistance, and targeted therapeutic medical applications.In the decades since their invention, hundreds of thousands of CO2 lasers have been used in medicine, manufacturing, and scientific research-from printing a four-digit code on water bottles in a high-speed manufacturing line in China to welding component parts for Mercedes-Benz automobiles in Germany. Even today, with fiber lasers gaining popularity in similar applications and new frontiers being forged by quantum-cascade lasers (QCLs), CO2 lasers will remain one of the most widely deployed lasers on the market if they can progress into application-specific territory.
Challenging the competition
Despite these long-time advantages, CO2 lasers are being challenged on several fronts. Fiber lasers and QCLs have migrated into some of the applications formerly dominated by CO2 lasers.
For industrial applications, high-power fiber lasers can provide higher efficiency and better absorption by metal at a cheaper or comparable cost. However, CO2 lasers remain the only way to process many non-metal materials that do not absorb the NIR wavelengths provided by fiber lasers.
Quantum-cascade lasers are also compact in size and can produce wavelengths spanning 2–12 μm, making them a great tool for spectroscopy. However, many sensing and spectrum-sensitive industrial and medical applications in the long-wave infrared (LWIR) region from 8 to 12 μm require a combination of high power, spectral purity, excellent coherence, and stable spatial mode that only CO2 lasers offer.
In addition to technological challenges, price erosion—primarily from China's ever-expanding laser industry—has pushed prices lower and lower. Standard CO2 lasers are becoming a pure commodity, with barriers to entry and margins dropping rapidly. Just three years ago, Chinese companies were purchasing U.S.-made 30 W CO2 lasers for $4500. Now, Chinese laser manufacturers have entered the market with their own CO2 lasers for $2000.
These factors signal an end to the "dollar-per-watt" era, wherein companies produced lasers with a specified average power, the capability to perform a myriad of tasks, and a price proportional to the watts delivered. Using this strategy, highly successful companies like Synrad, Coherent, and Rofin created families of lasers ranging from a few watts to tens of kilowatts, birthing an industry where CO2 lasers were used in plastic manufacturing plants, dentists' offices, and cell phone assembly lines.
Although the ability of CO2 lasers to compete as a one-size-fits-all solution is coming to an end, we are meeting the challenges posed by the emerging world of new materials and increasingly demanding industrial and scientific processes with a new application-specific lasers paradigm that requires a much deeper technical understanding of the true value proposition of a laser, and a different approach to how CO2 lasers are manufactured and marketed.
On the manufacturing side, this new paradigm leverages the wide-ranging specifications of CO2 lasers to closely match specific customer requirements. On the marketing side, it shifts away from average power and dollars-per-watt as the dominant value proposition towards customer-specific solutions where pulse shaping, peak power, wavelength specificity, and operational stability match specific material and applications needs.
Enabling EUV lithography
In the attempt to extend Moore's Law, CO2 lasers have been identified as the optimal tool to generate extreme ultraviolet (EUV) radiation through laser-produced plasma (LPP). This EUV light at 13.5 nm is produced by vaporizing molten droplets of tin. Other lasers such as neodymium yttrium-aluminum-garnet (Nd:YAG) were tested for this application, but proved less efficient than CO2 lasers that generate optically thin plumes with higher velocities and better plasma properties because of tin's much higher reflectivity to CO2 laser illumination.2
To generate EUV light, CO2 lasers are required to provide a stream of high-speed identical pulses with virtually perfect optical quality at a single wavelength (10.6 μm)—all with the cleanliness, accuracy, and repeatability required by the semiconductor industry.
To meet these exacting requirements, CO2 lasers for EUV are completely manufactured in an ISO Class 7 cleanroom, from processing raw parts through to final test (see Fig. 2). Every laser passes a continuous 12-hour output test, holding perfectly to the test specification, as any deviation could create thousands of failed semiconductor chips.Removing LIGO distortion
Our decade-long collaboration with the Laser Interferometer Gravitational-wave Observatory (LIGO) project provided critical technology in the quest to confirm the presence of gravitational waves. Although Einstein had predicted their existence long ago, their theoretical signal was so small that he was skeptical that an apparatus sensitive enough to detect these faintest murmurs of the universe could be built.
The scientists at LIGO contacted us with a very specific problem. Any photons absorbed by the interferometer optics, even one out of 3.3 million, distorted the optics and created enough thermal noise to bury the signal they were trying to detect. Although LIGO had designed their optics to take thermal distortion into account, in practice they needed to fine-tune them in real time to match the power of the main beam and the individual quality of each optic.
Carbon-dioxide lasers were the logical way to tune the optics because their beams are heavily absorbed by LIGO's optics and they can provide high wavelength purity and power stability, without which they would have only added more noise to their measurements.
But by working with LIGO's exact application-specific needs, we developed a single-wavelength-stabilized CO2 laser suitable for a very precise control loop within a real-time adaptive system that precisely countered the optical distortion because of heat from the main working beam (see Fig. 3).3 When the optics were under-compensated for thermal distortion, the CO2 laser beam was shaped into a ring to provide additional compensation (over-heat correction), whereas if the optics were over-compensated, the CO2 laser beam was shaped into a disk in the center of the optic to weaken the compensation (under-heat correction).Dental surgery
Recently, scientists discovered that human hard tissues (bones and teeth) have strong absorption in the 9.3–9.6 μm range (see Fig. 4).4-6 Studies have also shown that CO2 lasers are uniquely suitable for oral surgery on both hard and soft tissue, and for preventing dental caries (tooth decay).Although other wavelengths, such as 2.94 μm, are found to work well with soft tissue and hard tissue alike, only CO2 lasers at 9.3 and 9.6 μm wavelengths provide the additional benefit of caries prevention and removal.7 Professor John Featherstone, dean of the UC San Francisco Dental School, likened the discovery to the sun rising in a new era of laser dentistry.8
With pulse energies of multi-millijoules, pulse lengths of 5–20 μs, and thousands of hours of reliable operation, CO2 lasers uniquely suit dental surgery and treatment in a point-of-care setting. Although this technology is far from achieving mass adoption, commercial dental systems based on 9.3 μm pulsed CO2 lasers are now available for hard and soft tissue applications.9
Processing new materials
As manufacturing continues its global shift towards full process automation, lasers are changing the value proposition for traditional processes such as knife cutting and punch pressing of materials in industries like packaging. Companies such as Amazon are driving a change from rigid packaging—set sizes of traditional cardboard boxes—to flexible packaging constructed from new plastic materials and created onsite to exactly fit the needs of the particular shipment.
Flexible packaging is lighter in weight and safely biodegradable in a landfill, reducing the fixed costs and environmental impact of shipping and packaging—key future performance metrics for Amazon. This shift is enabled by the fact that lasers never go dull and deliver repeatability and accuracy well beyond the capabilities of mechanical systems.
New types of multilayer plastics can have a very strong absorption peak at one particular line in the CO2 spectrum, or even require a laser that can shift wavelengths on the fly as it goes from layer to layer in the cutting process. Replacing a large-form knife-cutting system would require an array of lasers capable of creating ablation with almost no heat-affected zone, while tracking a web moving hundreds of feet per second. This application-specific laser needs high accuracy at a single wavelength in the spectrum, with potentially very high peak-power-to-average-power ratios of 100 to 1 or even higher, and very fast (>10 kHz) pulses.
Traditionally, such peak powers and fast pulses require Q-switching or other external modulation techniques that are simply too expensive to scale for cost-effective mass deployment in industries such as packaging. However, we are developing CO2 lasers that achieve kilowatt peak powers without external modulation in a small form factor to meet these demands. Indeed, the newest trends in manufacturing, medicine, and materials science are forcing the historical dollar-per-watt value proposition of the last half-century to evolve into a customer-centric, application-specific era for the CO2 laser.
REFERENCES
1. C. Freed, LLabJ, 3, 3, 479–500 (1990).
2. A. Hassanein, V. Sizyuk, S. S. Harilal, and T. Sizyuk, "Analysis, simulation, and experimental studies of YAG and CO2 laser-produced plasma for EUV lithography sources," Proc. SPIE, 7636, 76360A (2010).
3. S. Ballmer et al., "Thermal compensation system description" (2005); see http://bit.ly/2dwiUNf.
4. P. Rechmann et al., Lasers Surg. Med., 45, 302–310 (2013).
5. N. M. Fried and D. Fried, Lasers Surg. Med., 38, 335–343 (2001).
6. D. Fried et al., J. Biomed. Opt., 6, 2, 231–238 (2001).
7. S. Parker et al., J. Laser Dentistry, 20, 2, 46–51 (2012).
8. J. Featherstone, Invited speech at the Annual Conference of Academy of Laser Dentistry (2013).
9. See http://www.convergentdental.com.
Yong Zhang is president and chief technology officer and Tim Killeen is sales and marketing manager, both at Access Laser, Everett, WA; e-mail: [email protected]; www.accesslaser.com.