Solid-state Lasers: Cascaded, multi-harmonic generation yields all-solid-state deep-UV emission
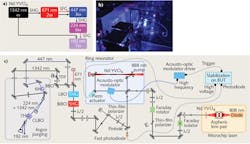
PETER KOCH, JÜRGEN BARTSCHKE, and JOHANNES A. L'HUILLIER
Coherent radiation in the deep-ultraviolet (DUV) spectral range, with a wavelength of less than 200 nm, is of great interest for several applications, such as lithography, metrology, spectroscopy, and the fabrication of fiber Bragg gratings (FBGs). Most of these applications benefit from a narrow linewidth and a diffraction-limited beam profile. For example, using an interferometric inscription technique, the maximum length of a FBG is limited by the bandwidth of the inscribing laser because of self-apodization.
While there are no solid-state laser materials available that provide direct laser emission in the DUV spectral range, argon-fluoride (ArF) excimer lasers provide direct emission at 193 nm at output power levels of more than 100 W, dominating high-power applications such as lithography.
Conversely, all-solid-state DUV light sources offer advantages for low-power applications like metrology and the fabrication of FBGs. These all-solid-state sources are realized by frequency conversion of infrared (IR) lasers and provide superior beam quality and excellent spectral properties compared to ArF excimer lasers, but their realization is still challenging. For metrological applications in the semiconductor industry, such as characterization of complex stepper optics, an emission wavelength of 193.368 nm and a narrow bandwidth are imperative. However, for the fabrication of FBGs, any wavelength in the DUV spectral range below 200 nm is sufficient.
IR conversion
All-solid-state DUV sources are generally based on cascaded second-harmonic generation (SHG), sum frequency generation (SFG), and difference frequency generation (DFG) of IR solid-state lasers. So, the possible concepts—consisting of the choice of fundamental wavelength and the conversion scheme—are defined by the materials that provide phase-matching and transparency in the DUV spectral range.
Readily available nonlinear crystals that provide these necessary properties include barium borate (BBO), lithium triborate (LBO), lithium tetraborate (LTB), cesium lithium borate (CLBO), and more recently, potassium fluoro-beryllo-borate (KBBF). However, because of its plate-like structure, only few-millimeter lengths of KBBF crystals are available. Therefore, KBBF is mostly used for the efficient conversion of picosecond and femtosecond pulses, and is insufficient for effective conversion of narrowband nanosecond pulses.
Because of the low birefringence of BBO, LBO, LTB, and CLBO, DUV radiation below 200 nm can only be obtained by SFG of UV sources above 200 nm using visible or IR radiation with these materials. And because LTB offers only a small nonlinearity, most narrowband all-solid-state DUV sources use BBO, LBO, or CLBO in the last sum frequency mixing stage.
Previously, DUV light sources based on titanium sapphire (Ti:sapphire) master oscillators and power amplifiers, fiber amplifiers, and 1 μm neodymium (Nd) lasers, or a combination of those, have been demonstrated. Because of the reliability and maturity of Nd laser technology, many approaches for an all-solid-state frequency conversion chain to the DUV start with a fundamental wavelength of 1064 nm. However, sum frequency mixing to the DUV between 190 and 200 nm requires a parametric conversion stage, which limits the efficiency and necessitates bandwidth narrowing.
DUV by seventh-harmonic generation
An attractive alternative to reach the DUV spectral range below 200 nm is the cascaded SHG and SFG of 1342 nm neodymium yttrium vanadate (Nd:YVO4) lasers up to the seventh harmonic at 191.7 nm. This is especially interesting for the fabrication of FBGs, which does not require a specific wavelength in the DUV spectral range.
In this conversion scheme, there are four conversion stages that generate the second harmonic at 671 nm in bismuth borate (SHG in BiBO), the third harmonic at 447 nm by sum frequency mixing the second harmonic with the fundamental (THG in LBO), the sixth harmonic at 224 nm by frequency doubling the third harmonic (6HG in BBO), and finally the seventh harmonic by sum frequency mixing the sixth harmonic with the fundamental (7HG in CLBO) to create a 191.7 nm DUV light source (see Fig. 1).
The first two conversion stages use non-critical phase matching, allowing use of long crystals without the detrimental effects of walk-off and limited-angle-acceptance bandwidth. These cascaded, high-harmonic generation methods have allowed our team at Photonik-Zentrum Kaiserslautern to demonstrate both a broadband and a single-longitudinal mode DUV source.
Broadband DUV
The broadband setup was based on a multi-longitudinal mode Q-switched 1342 nm Nd:YVO4 laser that provided an average power of 15.2 W and a pulse duration of 16.3 ns with a pulse repetition frequency of 10 kHz and a spectral width of 29 GHz.1 The laser had very low pulse energy fluctuations of <1% and diffraction-limited beam quality (m2 <1.1).
The frequency tripling stage was optimized by exploiting the nonlinear phase shift of a phase-mismatched SHG process.2 In principle, this phase shift is caused by the superposition of the non-converted fundamental with the fundamental mode, which is generated by back conversion of the second harmonic. This nonlinear phase shift is the reason for Kerr-like self-action of the fundamental beam. With this self-action, the beam diameter of the second harmonic and the fundamental in the THG crystal could be matched, which improves the beam quality of the third harmonic significantly. By detuning the phase-matching temperature of the SHG crystal, the conversion efficiency from the fundamental to the third harmonic as well as the beam quality of the third harmonic is maximized by optimizing the power ratio of the fundamental to the second harmonic and the spatial overlapping.
We used a polarization bypass in front of the SHG stage to bypass a part of the 1342 nm power to avoid the usage of the residual 1342 nm beam in the last conversion stage. In contrast to the residual beam, the bypassed 1342 nm beam has excellent beam quality, which improves the performance and the beam quality of the 7HG conversion stage. For a bypass of 38%, a power of up to 240 mW at 191.7 nm has been demonstrated—corresponding to an overall efficiency of 1.8%. At a total pump power of 9.45 W after the variable attenuator, we obtained a power of 190 mW (2% overall efficiency) with a Gaussian-shaped beam profile and m2 <1.9.
An even-better beam quality of m2 <1.7 is demonstrated with a bypass of 25% and a total pump power of 8 W, resulting in 155 mW at 191.7 nm. The pulse duration of the seventh harmonic is 12.1 ns and the pulse energy fluctuations are quite high (<10%) because of the multi-longitudinal-mode operation.
Single-mode DUV
Our single-mode DUV setup is based on an injection-locked, Q-switched 1342 nm Nd:YVO4 ring laser with an average power of 13.9 W and 18.2 ns pulse duration at a pulse repetition frequency of 10 kHz.3 The pulse energy fluctuations are very low (<0.6%) and the beam quality is m2 <1.05. The seed laser is a continuous-wave (CW) single-frequency Nd:YVO4 microchip laser and the ring resonator is stabilized with the build-up time (BUT) method.
At the second harmonic, the single-mode Q-switched laser exhibits a spectral width of 45 MHz during a measurement time of 11 ms and a long-term spectral width of 75 MHz that is broadened by vibrations and the modulation of the cavity length for the stabilization via BUT reduction. The experimental setup for the conversion to the DUV spectral range is an optimized version of our broadband setup. The modulation of the cavity length for the stabilization via BUT reduction and the experimental setup for the conversion to the DUV spectral range is an optimized version of our broadband setup.Finally, our DUV source is characterized by a homemade scanning confocal Fabry-Perot interferometer (FPI) with a free spectral range of 500 MHz (see Fig. 3). For a measurement time of less than 10 ms, the spectral width is less than 100 MHz full width at half maximum (FWHM). Adding in vibrations and frequency jitter associated with resonator stabilization, long-term spectral width values are on the order of 240 MHz FWHM. Because of its high coherence at 191.7 nm, our DUV source is well suited to the inscription of long gratings in a FBG production line.
REFERENCES
1. P. Koch et al., Opt. Express, 22, 11, 13648–13658 (2014).
2. P. Koch et al., Opt. Express, 23, 10, 13648–13658 (2015).
3. P. Koch et al., Opt. Express, 23, 24, 31357–31366 (2015).
4. P. Koch et al., Appl. Opt., 55, 8, 1871–1877 (2016).
Jürgen Bartschke is managing director of Xiton Photonics, Kaiserslautern, Germany; www.xiton-photonics.com, while Peter Koch is scientific researcher and PhD candidate and Johannes A. L'huillier is managing director at Photonik-Zentrum Kaiserslautern, Kaiserslautern, Germany; e-mail: [email protected]; www.photonik-zentrum.de.