Fiber Lasers: Single-crystal fibers amplify power in ultrashort-pulse lasers
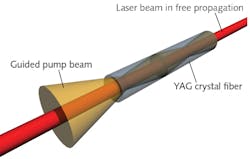
DANIEL GUILLOT, JULIEN DIDIERJEAN, and PATRICK BEAURE D'AUGÈRES
In laser microprocessing applications, short pulses deliver precision, high average power enables higher processing speeds, and high energy provides improved processing throughput. Short pulses can be readily achieved, but scalability—in terms of energy or average power—is more challenging. For example, nonlinear effects within optical fiber limit the peak power during amplification.
A well-accepted solution for achieving higher power in a short-pulse-width or ultrashort-pulse (USP) laser is through the master oscillator power amplifier (MOPA) architecture using various techniques and materials to affect amplification. The next generation of USP lasers depends on novel fiber-based architectures to overcome the shortfalls of traditional bulk and fiber amplifiers.
Amplification comparisons
Bulk-crystal amplifiers suffer from beam degradation because of the strongly aberrant thermal lensing effect created inside the gain media. With this fundamental limitation, high-power amplifier geometries have evolved to improve heat management inside these materials.
Thin disks deliver limited gain per pass because of the small thickness (approximately 100 μm) of the gain medium. As a consequence, effective amplification can only be achieved through multipass or regenerative techniques.
Multipass amplifiers use an elaborate optical path to pass the light through the laser crystal many times, while regenerative amplifiers use expensive electro-optic modulators as part of a complex beam-switching procedure. Despite the drawbacks, thin-disk amplifiers can generate very high kilowatt-level output powers and are ideal as final amplification stages.
While very high average powers—up to the kilowatt level—can be achieved in slab amplifiers because of their high gain, the zig-zag optical path used can introduce ellipticity and degrade beam quality.
Beyond bulk alternatives for creating short pulses, optical-fiber-based amplifiers typically use large mode area (LMA) fibers or photonic crystal fibers with large mode area (PCF LMA), while rod-type PCFs can achieve even higher peak powers.
Commercially available fiber has a maximum core diameter of 85 μm, a propagating mode of 65 μm, and, even though the doping level is high, a length of around 1 m. The very large core and extremely low numerical aperture of the fiber design delivers a diffraction-limited beam, but leads to inflexible and bulky systems.
The overlap between the guided pump and the guided laser mode yields good optical efficiency. Average power up to 200 W with good beam quality can be achieved—although at higher powers, thermo-optical effects can significantly influence the waveguiding mechanism. These perturbations cause LMA fibers to also support higher-order modes at high power, which leads to beam quality degradation and eventually to output beam fluctuations on the millisecond time scale (transverse mode instability). In industrial laser systems, the peak power in rod-type fibers is typically limited to around 1 MW to avoid nonlinear effects.
In femtosecond fiber lasers, chirped-pulse amplification (CPA) alleviates this limitation and high-power systems based on 40-μm-core flexible PCF fibers are available. However, the peak power limit forces use of large stretching ratios with corresponding compressors, increasing the cost and system size.
Single-crystal fibers
A single-crystal fiber (SCF) is usually monocrystalline yttrium aluminum garnet (YAG) with a long length, a small diameter, and attractive light-guiding properties.
Laser-heated pedestal growth (LHPG) techniques can produce small-diameter fibers (around 100 μm) and, through continual improvement, the goal is to achieve a core/clad structure similar to classic fibers for high-average-power laser systems. However, the production of such a structure is a difficult challenge that is far from being achieved, particularly when maintaining linear polarization is required. In spite of the interaction length decrease compared to classical fibers, the resulting architecture would, in any case, still suffer from the same limitations as silica fibers for high-peak-power pulses, and these would be aggravated even further by the higher nonlinear properties of the YAG material. Clearly, this is not the solution for ultrafast high-power amplifiers.
Larger SCFs of 1 to 2 mm in diameter have been produced using a micro-pulling-down technique that can sustain megawatts of peak power, making them ideal candidates for high-energy short-pulse amplification.
SCF amplifiers
The preferred configuration for amplification uses 1 mm diameter neodymium- or ytterbium-doped YAG SCFs about 30-50 mm long. The latter Yb:YAG medium is preferred for USP laser systems because of its larger absorption and emission bandwidths, longer upper-laser level lifetime, and lower thermal loading per unit pump power.
Commercial SCF amplifiers, such as the Taranis laser gain module, use a specific pumping scheme: the pump beam is focused to a 400-μm-diameter spot inside the crystal (rather than on its surface) and deep enough to maximize the amplifier output. The highly divergent beam is then guided by total internal reflection and refocused again in the gain medium (see Fig. 1).
The initial focusing point of the pump is imaged several times along the fiber length, depending on the pump brightness. For superior beam quality, the pump beam is kept collinear to the laser beam during the propagation in the gain medium, thus avoiding off-axis aberrations such as astigmatism that can appear in slabs or thin disks.
The doping level is typically one order of magnitude lower than in bulk crystals, and the length typically one order of magnitude longer. Without pump guiding, these differences would be unproductive because of the high divergence of the pump. Yet with pump guiding, this long-length/low-doping-level ratio spreads the pump absorption along the entire length of the crystal fiber and therefore lowers thermal stress in the gain medium.
Single-crystal fibers in a MOPA configuration have already proven their efficiency and flexibility in the field. In practical terms, Yb:YAG SCF gain modules produced by Fibercryst were pumped up to 600 W at 940 nm without damage—far above the typical pumping level of usual bulk crystals.1
Cooling a 1-mm-diameter YAG rod subjected to hundreds of watts of pump power is not easy, but is a feat accomplished by the Taranis module—a technology patented by Fibercryst and the CNRS Laboratoire Charles Fabry (LCF) to integrate the crystal fiber directly into a metallic mount. Cooling efficiency is very high, with a thermal exchange coefficient up to 5 W/cm2/K—five times better than typical indium-pressed solutions (see Fig. 2).The thermal gradient in the pumped crystal fiber is radially symmetric and guarantees excellent beam quality, even at high power. A high average power of 140 W has been demonstrated, without reaching the limit of the SCF technology.2
High average power and high energy
In a rod-type amplifier, the typical surface of the laser mode is 3000 μm2. Nonlinear effects, including self-phase modulation (SPM) or Raman interference, quickly appear when high peak intensities are propagated in such small surfaces with a long length of interaction.
In a typical SCF amplifier, however, the surface covered by the laser mode is 50X higher and, as a consequence, substantially higher peak powers can be achieved. The combination of high gain, small length, and large beam diameters have already demonstrated small signal gains of around 30 dB and peak powers up to 50 MW.
As an improved "bulk crystal" amplifier, SCF technology keeps all the advantages of usual bulk amplifiers—especially their ability to operate independently over a large range of repetition rates, pulse durations, and seed powers. The same SCF amplifier can be used, with no adjustment, to amplify a pulsed seed laser from a few kilohertz to ≥20 MHz, or a seed laser from tens of nanoseconds down to a few-hundred femtoseconds. Seed powers amplified from a few hundred milliwatts up to tens of watts have already been successfully demonstrated (see Fig. 3).3,4A typical industrial single-pass Yb:YAG SCF amplifier stage only has six optics sets. This compares favorably with disk or slab technologies.
SCF amplifiers have been used with a variety of seed lasers to display the flexibility and simplicity of the setup. For example, we report an architecture based on SCF associated with a divided pulse amplification (DPA) setup dedicated to high energy by providing 6 ps pulses with energy of 2 mJ at the repetition rate of 12.5 kHz.
For high average-power applications, a system delivering 100 W without CPA has been obtained with a 20 MHz repetition rate and a 750 fs pulse width.5 In both configurations, output beam quality excels with m2 values below 1.2.6
Using SCF amplifiers in a cascade and a femtosecond fiber-based seeder, the Fibercryst FEMTO25 industrial laser has a very large envelope of operation with excellent m2 and ellipticity. The energy per pulse of this laser can be changed instantly for process development, and the overall modularity of the laser adapts to any application.
REFERENCES
1. X. Delen et al., Opt. Lett., 37, 14, 2898 (2012).
2. S. Piehler et al., Opt. Express, 21, 9, 11376 (2013).
3. I. Martial et al., Opt. Express, 19, 12, 11667 (2011).
4. X. Delen et al., Opt. Lett., 38, 2, 109 (2013).
5. F. Lesparre et al., Opt. Lett., 40, 11, 2517 (2015).
6. F. Lesparre et al., Opt. Lett., 41, 7, 1628 (2016).
Daniel Guillot is CEO, Julien Didierjean is operations manager, and Patrick Beaure d'Augères is laser R&D engineer, all at Fibercryst, Décines-Charpieu, France; e-mail: [email protected]; http://fibercryst.com.