PHOTONIC FRONTIERS: BEAM COMBINING: Beam combining cranks up the power
Fiber and diode lasers can generate impressive powers from small volumes, but nonlinear losses, thermal effects, and optical damage limit the output from a single laser aperture. Combining the outputs of many laser apertures can overcome those limitations.
The simplest approach is incoherent beam combining, which directs many laser beams in the same direction, increasing total power but not increasing the beam brightness. More sophisticated techniques can raise brightness. One is combining beams coherently, so their amplitudes add constructively. An alternative is combining beams of different wavelengths, as in wavelength-division multiplexing, which avoids the complexities of phase matching but produces wider-band laser emission. Both approaches have produced markedly higher powers since Laser Focus World last reviewed the field four years ago.1 But so far, incoherent beam combining still holds the power record and is under development for laser weapons.
Incoherent beam combining for raw power
Monolithic arrays of semiconductor diode lasers are the most established example of incoherent beam combining. The beams from many laser stripes combine in a broad high-power beam that is not strongly directional but is valuable for laser pumping and applications such as heat treating that do not require high directivity.
Incoherent combination of the high-quality beams from fiber lasers can generate much more directional beams that are getting serious consideration for use as laser weapons. "Beam brightness at the source is of limited importance when considering realistic [directed-energy] propagation scenarios in turbulent atmospheres," wrote Phillip Sprangle of the Naval Research Laboratory (Washington, DC) and colleagues in 2008.2 Fiber laser arrays are living up to that promise. The US Navy assembled an array of six 5.5-kW industrial fiber lasers to produce the 33 kW Laser Weapon System (LaWS), which in 2010 engaged representative targets over the water at a distance of one nautical mile. Encouraged by those tests, the Navy is scaling LaWS to the 100 kW range, with completion targeted in 2014.3
Diode coherent beam combining
Coherent beam combining resembles phased-array radar—an array of emitters transmitting in phase to generate a coherent output. In principle, operating the emitters in phase and combining their beams properly can generate a steerable beam, but so far most effort has gone to combining the outputs.
Diode coherent beam combination was demonstrated with up to 900 laser stripes in the 1990s, but the need for singlemode output and tight phaselocking requirements limited output to 5.5 W. New techniques and the use of master-oscillator sources to drive many parallel amplifiers have overcome those limitations. Last year, Tso Yee Fan and colleagues at MIT Lincoln Laboratory (Lexington, MA) produced 38.5 W continuous wave from an array of 218 individually addressable slab-coupled-optical-waveguide (semiconductor) amplifiers (SCOWAs).4
First, a diffractive optical element split the seed laser beam into 21 outputs, and delivered them to parallel amplifiers with large circular mode profiles on a 200 μm pitch, as shown in Fig. 1. A double-pass through the amplifier array produced 21 parallel beams, which were monitored to generate phaselocking signals. Then a second diffractive optical element, aligned orthogonally to the first, divided each of those 21 parallel outputs into 11 parts, one for double-pass amplification of each of 11 parallel SCOWA arrays. That produced phaselocked output from 218 of the 231 stripes in the second-stage SCOWA arrays; the remaining 13 stripes did not work. Phase control over that large number of elements was crucial for success. "We're trying to push how many elements we can control," says Fan. The fundamental limits are not clear, he adds; "one way to prove how far you can go is experiments."
Diode wavelength beam combining
Wavelength beam combining (WBC) can generate much higher powers and brightness levels from diode lasers; in January 2012, TeraDiode (Littleton, MA) reporting reaching 2 kW at Photonics West.5 "That demonstration shows that direct diodes can produce the same kind of brightness as the best fiber lasers, making them suitable for industrial applications," says Parviz Tayebati of TeraDiode. Moreover, the intrinsic wall plug efficiency is quite high, reaching 46% for recent prototype systems.
Their approach is based on the external cavity design shown in Fig. 2, which places a diode array within an external cavity containing a transform lens, a diffraction grating, and an output coupler. The external cavity optics are aligned to select a different oscillation wavelength in each laser stripe, closely spaced across the diode's 40 nm gain band centered at 970 nm. The angles select and stabilize the wavelength. The combined output has a bandwidth of about 30 nm and can be coupled into free space or into a beam-delivery fiber.TeraDiode is now commercializing its WBC technology with the goal of beta availability in late 2012. The design is based on a multiwavelength module that can deliver about 500 W continuous wave and can be stacked together to generate powers to about 6 kW. "In a recent demonstration supported by DARPA we demonstrated 360 W with a Beam Parameter Product (BPP) of 0.6 comparable to singlemode fiber laser technology. Our product introduction will provide a more tame BPP of 2 to 8 as required by major cutting and welding markets," says Tayebati. Brightness levels are comparable to those of industrial fiber and CO2 lasers, and about 10 to 100 times that of standard high-power diode lasers.
Fiber laser beam combining
Diffraction-limited fiber lasers can reach multikilowatt powers, but further power increases are constrained by thermal limitations and nonlinear effects such as stimulated Brillouin scattering. Beam combining offers a faster and simpler route to higher powers. Beams can be combined incoherently by mixing fiber-laser outputs in large-core multimode fibers, producing continuous outputs to 50 kW. But coherent or wavelength beam combining promises the higher brightness and better beam quality needed for many applications.
A team from Lincoln Labs and Northrop Grumman Aerospace Systems (Redondo Beach, CA) have compared coherent and wavelength beam combination with commercial 500 W ytterbium-fiber lasers.6 Coherent combination was harder to implement because it requires phase control to within a small fraction of a wavelength, close path-length matching, narrow laser linewidth, and uniform polarization, they found, but it offers high spectral brightness and allows phase adjustments for beam steering or atmospheric compensation. Wavelength beam control is simpler to implement but produces a broader spectrum, so it is best suited for applications requiring raw power delivery where spectral brightness is not important.
Fiber laser beams can be combined coherently by tiling output of an array of phaselocked lasers (or amplifiers) across an aperture, or arranging transform optics and a diffractive optical element to fill an aperture with light from a more widely spread array of fiber emitters, as shown in Fig. 3. The Lincoln-Northrop group found that tiling concentrated 58% of the total power in the desired central lobe for inputs to 4 kW. In theory, tiling should increase the on-axis intensity by a factor equal to the square of the number of emitting elements, but they found that tiling with eight emitters increased only by a factor of 64, indicating beam combining efficiency of 78%.7 Using the same fiber laser array, feed laser, and phase-control system with the aperture-filling approach and a five-element diffractive optical element, they reported beam combining efficiency of 79% at maximum power of the five beams used.They also tested wavelength beam combining with six of the same fiber lasers used in the coherent tests, but with seed lasers at different wavelengths, five spaced 0.4 nm apart and the sixth offset by 0.8 nm. The optical configuration was similar to that shown in Fig. 2 for wavelength beam combination of diodes. With that arrangement they reported 2.51 kW output and 92% combining efficiency. All three experiments yielded near-diffraction-limited output.
Outlook for beam combining
Other developers are working on a wide range of variations on the theme of beam combining. At the University of Central Florida (Orlando, FL), Leonid Glebov has used volume Bragg gratings to coherently combine two fiber-laser beams with 99% efficiency to generate 282 W.8 Onera, the French Aerospace Laboratory (Palaiseau, France), last year coherently combined 100 ns pulses from a pair of fiber lasers with 95% efficiency, a challenge because nonlinear effects can exceed the bandwidth of the phaselocking controller.9 Coherent combination of beams from a pair of chirped-pulse fiber amplifiers has produced 3 mJ pulses with 89% efficiency.10
Progress is fast, and much remains to be sorted out. All three major approaches show promise, and each may find its own distinct applications. Coherent beam combining promises the best performance but appears the most complex approach. Wavelength beam combining may be easier to implement for many applications. And it will be interesting to see if incoherent combination is good enough for high-energy laser weapons.
REFERENCES
1. J. Hecht, "Photonic Frontiers: beam combining - Combining beams can boost total power," Laser Focus World, 44, 7 (July 2008).
2. P. Sprangle et al., "High-Power Fiber Lasers for Directed-Energy Applications," 2008 NRL Review, pp. 88–99; www.nrl.navy.mil/ content_images/08FA3.pdf.
3. R. O'Rourke, "Navy Shipboard Lasers for Surface, Air, and Missile Defense: Background and Issues for Congress," Congressional Research Service (Jan. 21, 2011); www.fas.org/sgp/crs/weapons/R41526.pdf.
4. S.M. Redmond, "Active coherent beam combining of diode lasers," Opt. Lett., 36, 909–911 (Mar. 15, 2011).
5. R.K. Huang et al., "Direct diode lasers with comparable beam quality to fiber, CO2, and solid state lasers," Proc. SPIE, 8241, 824102 (2012).
6. S.J. Augst et al., "Coherent and Spectral Beam Combining of Fiber Lasers," Proc. SPIE, 8237, 823704 (2012).
7. C.X. Yu et al., "Coherent combining of a 4 kW, eight-element fiber amplifier array," Opt. Lett., 36, 2686 (2011).
8. I. Divliansky et al., "Multiplexed volume Bragg gratings for spectral beam combining of high power fiber lasers," Proc. SPIE, 8237, 823705 (2012).
9. L. Lombard et al., "Demonstration of coherent beam combination of fiber amplifiers in 100ns-pulse regime," CLEO, paper CFE2 (2011).
10. A. Klenke et al., "Coherently combined CPA fiber laser system delivering 3 mJ femtosecond pulses," Proc. SPIE, 8237, 823708 (2012).
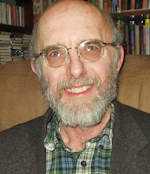
Jeff Hecht | Contributing Editor
Jeff Hecht is a regular contributing editor to Laser Focus World and has been covering the laser industry for 35 years. A prolific book author, Jeff's published works include “Understanding Fiber Optics,” “Understanding Lasers,” “The Laser Guidebook,” and “Beam Weapons: The Next Arms Race.” He also has written books on the histories of lasers and fiber optics, including “City of Light: The Story of Fiber Optics,” and “Beam: The Race to Make the Laser.” Find out more at jeffhecht.com.