SOLID-STATE LASERS: Laser pulsing: The nuts and bolts of Q-switching and modelocking
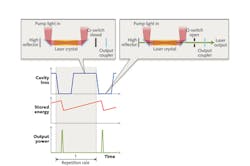
While there is a very broad selection of pulsed lasers currently available, the vast majority rely on just two pulsing techniques—namely, Q-switching and modelocking, able to deliver nanosecond or picosecond/femtosecond pulses, respectively. This article reviews the basics of each technology, the output characteristics that they deliver, and a few of the many applications that they support.
Q-switching
A Q-switch is essentially a shutter with a very fast response time placed inside a laser resonator. In one state (low Q) it creates cavity loss, preventing lasing; in the other (high Q), it has essentially no loss, so lasing can occur.
Key steps of the Q-switching process include the Q-switch state (cavity loss), energy storage, and output power as a function of time (see Fig. 1). At the start of each cycle, the Q-switching element is set to prevent lasing. While the Q-switch is in this mode, the pump energy, supplied continuously, is accumulated in the laser crystal (assumed to have a long upper-state lifetime). The Q-switch state is then changed, and the cavity loss quickly drops to zero. This causes a short pulse to build up by using all the stored gain. The combination of stored gain and short pulse rise and fall times results in a high peak power pulse with durations of a few nanoseconds up to a few hundred nanoseconds.
The most commonly used Q-switch devices are electro-optic (EO) and acousto-optic (AO) modulators. In an EO modulator, a crystal rotates the polarization of light passing through it when a voltage is applied. By inserting this crystal between two crossed polarizers, light can only pass through this assembly when the applied voltage rotates the polarization. EO modulators are typically used at low pulse-repetition rates (up to a few kilohertz) and high pulse energies, commonly in the range of multiple millijoules per pulse.
For higher pulse-repetition rates (tens of kilohertz or more) and lower pulse energies, an AO modulator is usually preferred. In an AO modulator, the crystal deflects the intracavity beam by a fixed angle when radio-frequency (RF) power is applied, thus preventing lasing.
Modelocking
Modelocking is a special operating condition of continuous-wave (CW) laser materials that is used for generating output with femtosecond or picosecond pulse duration. Laser materials with a wide gain bandwidth, such as Ti:sapphire, can produce a broadband output (tens of nanometers of more). Detailed examination reveals that this output consists of hundreds, thousands, or even tens of thousands of individual longitudinal modes, each satisfying the equation:
Nλ = 2 × cavity length (where N is a large integer)
In most lasers, these modes randomly gain and lose intensity, and have random phase relationships relative to each other. In modelocking, the relative phase of all these modes is fixed. Fourier analysis shows that the resultant superposition of modes manifests itself as a very short pulse that travels around the cavity at the speed of light. Every time this pulse reaches the output mirror, the laser emits a part of this pulse.
The shortest possible laser pulsewidth (called the transform-limited pulsewidth) ultimately depends on the number of modes involved. Since the pulse duration is the Fourier transform of the spectral bandwidth of the laser, broader bandwidths support shorter pulsewidths and vice versa. The pulse repetition rate is determined by the round trip time of the laser cavity. For example, in a Ti:sapphire laser oscillator with a cavity length of less than 2 m and a bandwidth greater than 100 nm, the repetition rate is 76 MHz and the pulsewidth can be close to 10 fs.
A CW laser can be forced into modelocked operation by any mechanism that provides lower optical losses when the laser runs in this regime rather than in a CW free-running regime. There are two categories of modelocking that can be used, called active and passive modelocking. Active modelocking refers to fast gating of the cavity (for example, using a AO modulator) at the cavity round trip rate, which is on the order of 76 MHz for a typical commercial Ti:sapphire oscillator.
Passive modelocking can be performed through the so-called Kerr lens effect in the gain material. Here the high peak intensity associated with modelocked operation induces a refractive-index change (equivalent to a lens) in the laser crystal. This lens focuses the beam, enabling it to pass through an internal aperture suffering only minor losses. In contrast, CW operation would result in clipping and attenuation. Another passive modelocking technique uses a "bleachable" optic, such as a saturable Bragg reflector (SBR). This is a special cavity mirror that only approaches 100% reflectivity when its absorbing semiconductor layer is overloaded by the high peak power of modelocked operation.
Q-switched pulses for materials processing
Efficient pulsing of a laser beam yields short pulses with high energy and high peak power. Q-switching is most commonly used with neodymium (Nd) doped laser hosts such as Nd:YAG, Nd:YVO4, and Nd:YLF. The dominant application for these diode-pumped, Q-switched lasers is in materials processing, and particularly precision micromachining, across most sectors of semiconductors/electronics and related industries (see Fig. 2). Q-switched diode-pumped solid-state (DPSS) lasers, such as the Coherent AVIA and Matrix series, provide ideal flexibility for these applications in terms of diversity of power, pulse repetition rate, and wavelength.Whether it involves drilling, cutting, or scribing, precision micromachining requires minimization of the heat-affected zone (HAZ), which defines the extent of any thermal damage to the surrounding material. The HAZ can be minimized by using shorter pulsewidths or shorter wavelengths. Shorter pulsewidths minimize the amount of heat that can escape and spread during the material-removal pulse, which is the reason that most Q-switched micromachining lasers are designed to produce pulses of a few tens of nanoseconds or less. Moreover, in some materials, shorter (ultraviolet) wavelengths enable material removal by direct bond breaking. The high peak output power of Q-switching is an advantage here since it enables efficient harmonic generation to convert the near-infrared (NIR; 1.06 μm) fundamental wavelength to 532 nm, 355 nm, or even 266 nm. Indeed, the overriding need to minimize HAZ means that NIR is used in only a few micromachining applications, such as marking, where they offer beam quality and stability advantages over fiber lasers.
Modelocked lasers now very diversified
The applications for modelocked lasers are very diverse, indeed far more so than for Q-switched lasers, and can only be briefly surveyed within the scope of this article. Moreover, these disparate applications are supported by similar diversity in available laser designs.Femtosecond Ti:sapphire oscillators are widely used as scientific research tools, often in conjunction with regenerative amplifiers, such as the Coherent Legend series that boosts the peak power to hundreds of gigawatts. These systems are used in applications such as generation of accelerated particles for high-energy physics, the production of intense terahertz pulses, time-resolved photochemistry, and even excising single genes in simple organisms. Moreover, cutting-edge femtosecond oscillators and amplifiers now feature optional control of the carrier-to-envelope phase (CEP)-the relationship between the pulse optical envelope and the phase of the oscillating electric field-enabling generation of attosecond x-ray pulses via high-harmonic generation (HHG).
In biology, compact, closed-box Ti:sapphire oscillators and wavelength extension accessories, such as optical parametric oscillators (OPOs), are now widely used as turnkey sources that enable nonlinear microscope imaging of live tissue. These imaging modalities include multiphoton excitation (MPE) and harmonic-generation microscopy (see Fig. 3).
A particularly high growth area is the use of ultrafast laser pulses for micro- and nanomachining. In particular, the combination of modelocked technology with fiber-laser technology has enabled a new generation of amplified picosecond lasers (such as the Coherent Talisker) that offer a choice of NIR, visible, and UV outputs. This type of laser is proving ideal for machining films of thin and/or delicate materials, since their short pulse duration ensures no HAZ. Current applications include various scribing processes for solar panels, patterning of organic LED (OLED) displays, cutting the next generation of medical stents, and precision drilling of thin glass for smart phone touchscreens, to name just a few (see article by C. Moorhouse, "Picosecond laser enables new high-tech devices," Industrial Laser Solutions, May 2012; http://bit.ly/JEWzZD).
In a quite different application, laser direct imaging (LDI) is a fast-growing technique for producing high-density multilayer PCBs. Here, the laser is used to directly write a pattern in a layer of photoresist. The application nominally requires tens of watts of CW or quasi-CW UV laser power in a low-noise TEM00 beam. This need is met by modelocked Nd:YVO4 lasers operating in the picosecond regime. The high peak power of modelocking enables efficient frequency tripling outside the laser cavity and delivers a repetition rate greater than 80 MHz, which appears completely CW even at the fast scan rate of this cutting-edge application.
The flexibility of Q-switching and modelocking enables the laser industry to provide a diverse selection of pulsed lasers for an ever wider range of scientific and industrial applications. This is possible because the versatility of these techniques allows them to be used in numerous laser types including DPSS, Ti:sapphire, and fiber lasers.
Marco Arrigoni
Marco Arrigoni is vice president of marketing at Light Conversion (Vilnius, Lithuania). He previously served as director of marketing at Coherent (Santa Clara, CA) from 2007 through 2023.
Magnus Bengtsson | Director of Strategic Marketing, Coherent
Magnus Bengtsson is Director of Strategic Marketing at Coherent (Santa Clara, CA).
Matthias Schulze | Director of Marketing, OEM Components and Instrumentation at Coherent
Matthias Schulze is Director of Marketing, OEM Components and Instrumentation at Coherent (Santa Clara, CA).