Photonic Frontiers: LEDs and other Light Sources: Looking Back/Looking Forward: More than lasers: LEDs, lamps, and other non-laser sources
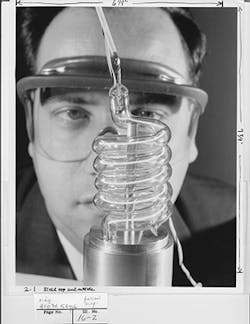
Laser Focus World has always focused on lasers, but it also covers other light sources. In the early days, those other sources generally had laser connections. Flashlamps pumped solid-state lasers. Lasers, in turn, pumped nonlinear sources such as optical parametric amplifiers and oscillators, sum-frequency generators, and supercontinuums. Incoherent LEDs were closely related to laser diodes, and their applications overlapped in early fiber-optic systems.
The distinctions between laser and non-laser sources have blurred over the years. Today's application engineers care less about how light is generated than they do about its behavior. Tunable OPOs compete head-to-head with lasers for some applications. Superluminescent diodes (SLEDs, or sometimes SLDs) beat laser diodes where low coherence is an advantage. So our coverage has evolved to follow our readers' interests and needs, and the expanding range of photonics technology.Flashlamps came first
A key to Theodore Maiman's success in making the first laser was pumping with a commercial photographic flashlamp. The smallest of the three coiled flashlamps made by General Electric, the FT-506, sufficed to power his little laser. But the photographer who took the press-release photo (see Fig. 1) thought the mid-sized FT-503/524 lamp looked better, and it also worked in efforts to reproduce Maiman's laser.Two water-cooled flashlamps from EG&G (then in Boston, MA) were among the new products in our first issue, January 1, 1965 (see Fig. 2). One fired 100 J pulses lasting 150 μs; the other 400 J pulses lasting 1 μs. Our December 15, 1965 product column (see Fig. 3) described a 10 kJ shock-resistant lamp from the Xenon Corporation (then in Watertown, MA). Laser pump lamps were the first product of the company founded the previous year in the family basement by three brothers—Lou, Gene, and Richard Panico. Lou had learned flashlamps from a master, the legendary Harold "Doc" Edgerton, the E in EG&G.
Shock resistance was an issue because flashlamps could explode when being charged. Industry veteran Bill Shiner recalls that he and Eli Snitzer kept two metal wastebaskets in their lab at American Optical to put over their heads when charging the lamps. "If the lamp failed, you'd hear the glass hit the wastebasket," he said.
A decade later, Xenon was developing mass production techniques for the thousands of flashlamps needed to build the 20-beam Shiva fusion laser at the Lawrence Livermore National Laboratory. The November 1974 issue described a new inner-seal design that Xenon said should cut costs, reduce shock-induced damage, and ease handling. A typical 20 kJ lamp used by Livermore then cost about $250, so building thousands was a big business.
LEDs, optical communications, and blue light
Demonstration of the first bright gallium-arsenide LEDs led to development of laser diodes in 1962. The first visible LEDs were made by Nick Holonyak in his quest for a laser diode. Visible LEDs soon found their first applications in indicator lights. The small size, brightness, and reliability of infrared GaAs LEDs made them alternatives to then short-lived diode lasers for early multimode-fiber links.In 1971, Charles Burrus of Bell Labs (Holmdel, NJ) invented an LED specifically for fiber systems, with a central hole etched through the metal contact and semiconductor to the emitting layer (see Fig. 4). The original design accommodated a step-index multimode fiber with 60 μm outer diameter.1 LEDs looked good for atmospheric and fiber communications, wrote Forrest M. Mims in our September 1974 issue: "The inexpensive LED, emitting up to 100 milliwatts CW at room temperature with drive current only about 100 amperes per square centimeter and with high modulation rates in the multimegahertz range, presents the strongest challenge" to laser diodes.
With their inherent immunity to electromagnetic interference, LEDs looked good for Navy avionic systems, typically spanning less than 100 m, we reported in February 1975. In February 1976, we reported LEDs powered "the world's first operational optical-fiber communications link," spanning several hundred meters for the Dorset police department in Britain to replace a copper cable destroyed by lightning. GTE chose LEDs for their first test of fiber carrying live telephone traffic, we reported in May 1977. However, as laser diodes improved, they became standard for transmission beyond a few kilometers. Today, LEDs are used mainly in short links and local area networks.
The 1980s saw a quest for bright short-wavelength LEDs, with major investments in zinc selenide and related II-VI compounds. Our June 1987 issue described experiments at the Universities of Durham and Manchester in Britain suggesting that metal-insulator-semiconductor structures might work better than conventional p-n junctions (see Fig. 5).
But the real breakthrough came from the taming of gallium nitride, long thought hopeless because of high defect levels. Isamu Akasaki showed a bright-blue LED at the Materials Research Society's fall 1991 meeting that he had developed at Nagoya University (Nagoya, Japan) with Hiroshi Amano. In 1993, Shuji Nakamura doubled conversion efficiency to 2.7% at Nichia Chemical (Tokushima, Japan), and his commercial LEDs had achieved long lifetimes by the time he demonstrated the first GaInN laser diodes, we reported in April 1996.
Although we focused on potential blue laser diode applications in HD videodisks, blue LEDs become the success story that earned Akasaki, Amano, and Nakamura the 2014 Nobel Prize. As we wrote in April 2001, combining blue GaInN LEDs with yellow phosphors opened the door to solid-state lighting. The U.S. followed Japan in launching a national lighting initiative, coordinated by the Optoelectronics Industry Development Association. The original 1999 proposal projected LED bulbs would generate 50 lm/W by 2010; I bought some rated at 48 lm/W in 2012. Solid-state lighting has made LEDs such a big business that it has its own PennWell publication, LEDs Magazine.
Adding aluminum to GaN increases the bandgap, and a February 2005 feature reported "the cutting edge in semiconductor light sources has moved into the ultraviolet." UV LEDs then emitted wavelengths to 240 nm, but practical LEDs could only reach 280 nm, and the limit of laser diodes was 370 nm.
Superluminescent diodes
SLEDs occupy a twilight zone between LEDs and laser diodes, with enough of a population inversion to produce stimulated emission but without the feedback to reach the laser threshold and oscillate. First demonstrated by Bell Labs in 1973,2 they have low temporal coherence and wide bandwidth, attractive for some applications. But they took time to become practical.
The development of optical coherence tomography (OCT) in the 1990s created a need for a broadband low-coherence source. Our March 2004 issue reported that superluminescent diodes were being used in commercial OCT systems. SLEDS soon proliferated.So did other applications. SLEDs sensed both oxygen and ammonia at the Indian Institute of Technology (Chennai, India), we wrote in December 2009. In May 2011 (see Fig. 6), we reported that University of Maryland (College Park, MD) had used SLEDs to generate truly random sequences of numbers by a physical process, attractive for applications ranging from gaming to secure key generation.
Nonlinear light sources
Pulsed lasers provided the high field intensity needed for nonlinear effects, as Peter Franken demonstrated in 1961 by frequency-doubling ruby laser pulses. Traditionally, low-order harmonics are considered a minor variation of laser light, but more complex laser-pumped nonlinear processes are not considered laser sources because their output is not stimulated emission.
Parametric frequency-conversion processes were well known from radio engineering, and theorists predicted optical counterparts in the early 1960s. The first demonstrations came in 1965. Charles C. Wang and George W. Racette reported the first optical parametric amplification at Philco Applied Research Laboratory (Blue Bell, PA).3
Two groups independently demonstrated the first optical parametric oscillators—Joe Giordmaine and Robert Miller at Bell Labs and Rem Khokhlov, Sergey Akhmantov, and Alexander Kovrigin at Moscow State University.Early experiments were difficult, and output was feeble. In September 1967 we described the first tunable parametric source, developed by Stephen Harris and his students M. K. Oshman and Robert L. Byer at Stanford University, which emitted about 10-12 W. Harris called its output, tunable between 540 and 660 nm, "not quite, but nearly as pure as laser light."4
Supercontinuum sources emerged from picosecond laser experiments at GTE Laboratories (Bayside, NY) in 1969. Robert Alfano and Stan Shapiro had set out to measure phonon lifetimes with a new picosecond laser. "When the laser first shot into a piece of glass, we saw something strange. We sent in green light, and it came out all colors," recalls Alfano. Thinking their spectrometer had a light leak, they taped over everything except the entry slit to block the stray light, but white light still exposed their photographic plates. Only when they replaced the plates with Polaroid color film did they realize they were producing a rainbow of color.
What was producing the colors? They first realized the rapid intensity variation in the picosecond pulse caused self-phase modulation. Further experiments showed self-focusing was producing filaments and dots that others had predicted. They also found four-wave mixing was converting two photons from the input pulse to a Stokes- and anti-Stokes-shifted pair in the supercontinuum, and detailed the results in Physical Review Letters.5 Supercontinuum sources continued to fascinate researchers for years. The cover story of our February 1977 issue (see Fig. 7) showed the target-shaped rainbow produced by firing 30 ps pulses into water in Nicolaas Bloembergen's lab at Harvard.
Meanwhile, some nonlinear sources started finding applications. In our February 1975 issue (p. 45), Chromatix advertised a "tunable laser system" with a Nd:YAG pump that spanned 225 to 3400 nm by including an OPO for the infrared, a dye laser for the visible, and harmonic generation for the ultraviolet. Our November 1976 issue reported Byer's plan for Raman-shifting the 10 μm output of a carbon dioxide laser to 16 μm for enrichment of uranium isotopes in uranium hexafluoride.
OPOs quietly found their way into military systems in the 1990s as tunable infrared sources that could deflect attacks on aircraft by heat-seeking missiles. Today, they are being challenged by quantum cascade lasers, but remain the dominant technology for directed-infrared countermeasures, I reported in an April 2014 feature.
"Developments [in parametric sources] over the last decade have been more in mechanical and optical engineering than in physics," Lucian Hand, president of Altos Photonics (Bozeman, MT), told me for a December 2014 feature. New systems are easier to use and can withstand temperature and vibrations so well they have been hauled up mountains to calibrate telescope sensors.
Research has benefited in other ways. The supercontinuum is a crucial building block for the femtosecond frequency comb, an exquisitely sensitive spectroscopic tool that earned John Hall and Ted Hänsch the 2005 Nobel Prize in Physics, as we reported in our December 2005 issue.
Present and future light sources
After half a century, flashlamp pumping is fading from the laser market. Traditional lamps are now facing competition from laser-driven plasma light sources like those made by Energetiq Technology (Woburn, MA) mentioned in our April 2011 issue. Focusing a CW laser diode beam into a bulb filled with xenon generates a 100 μm plasma spot, brighter and longer-lived than an ordinary lamp.
The future of semiconductor lithography may depend on extreme ultraviolet sources powered by pulsed carbon dioxide lasers zapping tiny droplets of tin to produce plasmas emitting at 13.5 nm, but that has been a difficult quest, as we reported in the June 2009 issue.
The invention of photonic crystal fibers has brought a revolution in nonlinear sources by allowing designers to control fiber properties such as dispersion and single-mode core diameter, Adam Devine and Anatoly Grudinin of Fianium wrote in a June 2013 feature. They noted an order-of-magnitude increase in supercontinuum power in the previous decade as well as broader bandwidth.
Manipulating dispersion is a major advance because it allows use of purely normal dispersion fibers, so you get pure self-phase modulation without solitons and other effects that can disrupt the supercontinuum, says Alfano. The new fibers can generate a supercontinuum from a tabletop titanium-sapphire laser, and several companies are selling supercontinuum sources. Alfano ruefully remembers not bothering to aim his picosecond laser into fibers being studied down the hall at GTE, saying "boy, did I blow it." But he was just being realistic with the technology available 45 years ago. Now he's excited by the prospects of using supercontinuums as broadband sources for brain and other biological imaging.
New microstructured fibers also open the door to fiber-based OPOs, we reported in a May 2014 feature. Fiber-based OPAs have also been demonstrated. Further improvements will increase the intensity available from broadband supercontinuum sources.
LEDs will have the broadest impact on society as a whole as they transform artificial lighting. By replacing incandescent and fluorescent light, they are reducing the cost and environmental impact of lighting. New combinations of red, green, and blue LEDs enable color-tunable lighting, for purposes from decoration to aiding sleep and improving work efficiency. And as LED technology evolves, we will find new, more attractive, greener, and more efficient ways to use it for illumination.
REFERENCES
1. Charles A. Burrus and B. I. Miller, Opt. Commun. V, 4, 307–309 (Dec. 1971).
2. T. P. Lee, C. A. Burrus, and B. I. Miller, IEEE J. Quantum Electron., QE-19, 820 (1973).
3. C. C. Wang and G. W. Racette, Appl. Phys. Lett., 6, 169–171 (1965).
4. S. E. Harris, M. K. Oshman, and R. L. Byer, Phys. Rev. Lett., 18, 732 (May 1, 1967).
5. R. Alfano and S. Shapiro, Phys. Rev. Lett., 24, pages 584, 592, and 1217 (1970).
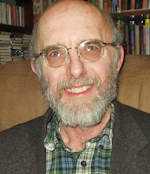
Jeff Hecht | Contributing Editor
Jeff Hecht is a regular contributing editor to Laser Focus World and has been covering the laser industry for 35 years. A prolific book author, Jeff's published works include “Understanding Fiber Optics,” “Understanding Lasers,” “The Laser Guidebook,” and “Beam Weapons: The Next Arms Race.” He also has written books on the histories of lasers and fiber optics, including “City of Light: The Story of Fiber Optics,” and “Beam: The Race to Make the Laser.” Find out more at jeffhecht.com.