QUANTUM-CASCADE LASERS: Optical illumination modulates quantum-cascade laser
Rapid modulation of the emission wavelength (optical frequency) of a mid-infrared (mid-IR) quantum-cascade laser (QCL) is difficult due to the nature of its parallel laser subbands and unipolarity; and although self-heating can cause a wavelength redshift, it cannot be used for high-speed modulation due to its thermal time constant, which is on the order of microseconds. But fortunately, researchers at the Stevens Institute of Technology (Hoboken, NJ), Chongqing University (Chongqing, China), and the National Research Council (Ottawa, ON, Canada) have developed a way to optically induce rapid wavelength modulation in a mid-IR QCL by illuminating its front facet with a near-IR femtosecond laser.1 Enabling wavelength modulation at frequencies up to 1.67 GHz, QCLs look even more attractive for environmental sensing and free-space optical (FSO) communications applications (see "Sophisticated optical systems extend reach of free-space communications," page 51, this issue).
Optically induced modulation
Wavelength modulation of a standard mid-IR QCL is enabled by directing an ultrafast Ti:sapphire laser at an angle of 30º to the normal of (and focusing it to a 20 μm spot size on) the front facet of the QCL. The 820 nm Ti:sapphire laser has a pulse duration of 100 fs and a pulse repetition rate of 83.3 MHz. In modulation terms, the 100 fs pulse train acts as a broadband-modulation frequency source beginning with its fundamental frequency at 83.3 MHz and including its higher harmonics up to about 10 THz.
The QC laser is a standard 35-period type indium-aluminum-arsenide/indium-gallium-arsenide (In0.52Al0.48As/In0.53Ga0.47As) Fabry-Perot QCL with a 7.6 μm central wavelength and a 2 μm thick core region with uncoated facets, mounted episide up on a copper heatsink. It is operated in continuous-wave (CW) mode at 400 mA.
Front-facet illumination with a 2 mW average-power pulsed Ti:sapphire laser generates an amplitude modulation of the mid-IR QCL at the repetition rate of 83.3 MHz. Next, a germanium etalon is placed in the collimated beam path of the mid-IR QCL between its two zinc-selenide lenses in order to convert an additional wavelength modulation into an amplitude modulation. The etalon, when rotated to the proper position, increases the magnitude of the amplitude modulation by more than 7 times, from 1.56 to 11.38 mV (an equivalent optical power of 7.5 μW). Because the etalon works as a narrow-bandpass filter, modulation can only be increased by emission-wavelength broadening or a true wavelength shift.
Proving wavelength shift
To directly prove that the modulation increase observed is due to a wavelength shift (true wavelength modulation), the QCL's CW spectrum and its 83.3 MHz modulated spectrum are compared at different incident power levels for the Ti:sapphire laser (0.5, 1.0, 1.5, and 2.0 mW). From this data, the observed wavelength shift is plotted against the incident power level, showing linear dependency of the modulation index on the near-IR power. That is, even though the amplitude of the modulated spectrum varies, the width of the CW spectrum remains constant, indicating that the modulation observed is due to a wavelength shift rather than a broadening of the laser emission.
The research team attributes the wavelength shift to two possible mechanisms. First, photon-generated free carriers in the QCL caused by the near-IR Ti:sapphire laser illumination induce a refractive-index decrease that effectively reduces the optical length of the cavity and causes a blueshift in the emission wavelength. Second, thermalization of the photon-generated hot carriers makes the part of the QCL near the front facet emit at a shorter wavelength, enabling very high modulation speeds. Measurements of the modulated QCL confirmed a modulation rate up to 1.67 GHz (see figure).
"The possibility to apply direct frequency modulation through optical illumination in addition to electrical amplitude modulation opens complete new modulation possibilities," says Rainer Martini, associate professor and director of the Laboratory at Stevens Institute of Technology. "We can now choose how data are transmitted and achieve high-quality and robust data transmission as we know from satellite links—only with much higher bandwidth. And this modulation technique may lead to also to a complete new way to coherently control and tune lasers."
REFERENCE
1. G. Chen et al., Appl. Phys. Lett., 97, 011102 (July 2010).
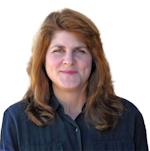
Gail Overton | Senior Editor (2004-2020)
Gail has more than 30 years of engineering, marketing, product management, and editorial experience in the photonics and optical communications industry. Before joining the staff at Laser Focus World in 2004, she held many product management and product marketing roles in the fiber-optics industry, most notably at Hughes (El Segundo, CA), GTE Labs (Waltham, MA), Corning (Corning, NY), Photon Kinetics (Beaverton, OR), and Newport Corporation (Irvine, CA). During her marketing career, Gail published articles in WDM Solutions and Sensors magazine and traveled internationally to conduct product and sales training. Gail received her BS degree in physics, with an emphasis in optics, from San Diego State University in San Diego, CA in May 1986.