PHOTONIC FRONTIERS: QUANTUM CASCADE LASERS: Quantum cascade lasers prepare to compete for terahertz applications
Users like Sheng Wu of the Power, Environmental, and Research Institute (Covina, CA) can testify to the dramatic progress in quantum cascade lasers (QCLs). When Wu bought his first in late 2005, driving the laser required a 7 A, 15 V pulse, yet he wondered if he was seeing blackbody emission rather than laser output. Just two years ago, a proposal reviewer scoffed at his idea of replacing "a cheap and reliable 10 W blackbody source used in every spectrometer" with a QCL emitting less than 10 mW "which might not even work continuously, barely tunes 1% of the infrared (IR), yet costs 100 times more." Now Wu is using mid-IR QCL spectroscopy to analyze carbon-isotope ratios in hydrocarbons.
Quantum cascade lasers are not just for research projects. In August, Block Engineering (Marlborough, MA) introduced three lines of QCL-based mid-IR spectrometers that the company says beat the performance of conventional Fourier-transform infrared (FTIR) spectrometers for near-field surface measurements, microscopy, and tunable sources (see Fig. 1).
Such improvements in mid-IR lasers and the growth of practical applications are two hallmarks of recent progress in QCLs, says Claire Gmachl, a Princeton University professor who heads the Mid Infrared Technologies for Health and the Environment (MIRTHE) consortium (Princeton, NJ). A third is the development of QCLs emitting in the hard-to-reach terahertz band.
Fundamentals
First demonstrated in 1994 by a team including Federico Capasso, now at Harvard University (Cambridge, MA), and Jerome Faist, now at the Swiss Federal Institute of Technology (Zurich, Switzerland), the QCL generates a quantum cascade of photons as electrons pass through a stack of quantum wells in a semiconductor.1 The electrons drop to a lower sub-band in the conduction band of the quantum well, then tunnel through to the next quantum well (see Fig. 2). A voltage is applied across the whole stack, so the same electrons can drop to lower energy levels repeatedly within different quantum wells in the stack.This process differs fundamentally from that in diode lasers, where electrons and holes recombine in a junction layer. Only electrons carry current in a QCL, and they pass unidirectionally through the stack of quantum wells, emitting a cascade of photons on sub-band transitions. Interband cascade lasers are hybrids of QCLs and diodes, with both electrons and holes passing through stacks containing junctions; they are being developed for wavelengths of 3 to 4 μm (see "Single laser emits beams at two angles," Laser Focus World, January 2010).
The two broad bands in which QCLs emit are called the "mid-infrared" and "terahertz," although they don't exactly match other definitions of those bands. For QCLs, mid-IR covers a range of 3 to 30 μm, much broader than the 3- to 5-μm band used in IR physics. Most QCLs are based on the alloys indium phosphide and gallium arsenide, which suppress emission at 30 to 60 μm by an effect called "reststrahlen" or residual rays. Terahertz QCLs emit at frequencies of 0.6 to 5.0 THz, corresponding to wavelengths from about 60 to 500 μm, but magnetic fields must be applied to generate frequencies below 1.2 THz.
Improving mid-IR performance and bandwidth
Like diode lasers, mid-IR QCLs have advanced beyond the first devices, which required cryogenic cooling and high drive currents for pulsed operation. Improvements include higher efficiency, continuous-wave operation at room temperature, broader wavelength range, higher power, and tunable output.
A key to improving QCL performance is improving electron transport, which requires studying both classical electron scattering and quantum tunneling within the devices. "We want to make the gain spectrum broader without compromising performance," says Gmachl.
Wavelength range is important for mid-IR spectroscopy, a major QCL application. Block Engineering's laser spectrometers use multiple QCLs to deliver 12 mW average power when scanning from 6-12 μm. Expanding the range to tune from 5-14 μm would give access to more gas bands, says Adam Erlich, vice president of marketing and business development. The system uses pulsed lasers that can operate at room temperature but are mounted on TECs to stabilize output power and wavelength. An advantage over FTIR systems is higher power in a narrow range of wavelengths, he says.
"Truly short-pulse quantum cascade lasers would be nice to have for applications," says Gmachl. Regular and controllable short pulses allow time-resolved measurements, but the gain dynamics of QCLs make conventional Q-switching and modelocking techniques impractical. Theorists are investigating alternatives that would work in QCLs, where gain recovers on a picosecond time scale. One possibility is trying to exploit the nonlinearities inherent in the system.
Power and applications
Milliwatts suffice for most spectroscopic applications, but QCLs have generated watts at wavelengths of 4-5 μm, sought for IR countermeasures to blind heat-seeking shoulder-launched missiles fired against aircraft. Pranalytica (Santa Monica, CA) offers a 2 W QCL emitting at 4 μm that is cooled only by a fan.
Improving efficiency creates a snowball effect, says Eric Takeuchi, director of business development at Daylight Solutions (San Diego, CA). "The more efficient you can make them, the more power you get out and the easier thermal management becomes." Daylight has shipped 5-10 W QCLs emitting at 4-5 μm for defense applications.
No other technology can generate such powers across the mid-IR, says Takeuchi. Quantum cascade lasers could be packaged with waveguide delivery systems for medical applications, he says. Tunable versions could allow surgeons to adjust wavelengths near water absorption peaks to control where power is delivered.
Today's products deliver diffraction-limited beams with 10-15% efficiency at room temperature. "In the next couple of years, we're going to be pushing 20-25%," says Takeuchi. "Three-watt output from a single chip in a diffraction-limited beam is definitely on the horizon." He also expects 8-10 μm powers to reach watts. "You're not pushing science results; you're working packaging systems for practical use."
Terahertz lasers
The first terahertz-band QCLs, reported in 2001, emitted only a few milliwatts at tens of degrees Kelvin.2 Rapid progress since then has pushed up operating temperatures and output.3 "There's some beautiful work coming out going well above 200 K," Gmachl says. "We're getting close to the thermoelectric cooling range," important because together with the small size of QCLs it offers the promise of a compact terahertz source.
Terahertz radiation is challenging because it often behaves more like microwaves than light. "The waveguides most often used in terahertz QCLs are more like microwave transmission lines," with metallic layers on top and bottom, than conventional optical waveguides, says Benjamin Williams of the University of California at Los Angeles. The waveguide is much thinner than the terahertz wavelength, so terahertz emission diverges strongly, spreading across 180°.
New techniques can confine emission to narrower ranges. Last year, Faist's group showed that fabricating a third-order waveguide along the waveguide could diffract the output into a beam diverging at 10°.4
This summer, Capasso's group reported that fabricating a metamaterial layer on the output facet could confine 100 μm emission of a QCL to a 10° cone.5 The heavily doped semiconductor acts like a metal at terahertz frequencies, so etching a series of micrometer-scale grooves into its surface formed a subwavelength metamaterial just below the end of the waveguide (see Fig. 3). Diffraction coupled the terahertz emission to the metamaterial, which radiated from an area large enough to limit divergence to 10°.Potential terahertz applications such as imaging, explosive detection, and local oscillators for radio astronomy generally require directional beams for ease of transmission, control, and focusing, says Nanfang Yu, a postdoctoral researcher who was lead author of the paper.
Outlook
Some practical applications for QCLs in the mid-IR will be military, in fields from remote hazard sensing to IR countermeasures. Civilian applications won't be far behind, and sciences such as chemical physics will benefit.6 As Erlich notes, the economics of the semiconductor industry, which drive prices down as volume rises, should help QCL systems compete with established mid-IR technologies. Also, QCLs can generate lines not readily available from other lasers, opening new possibilities.
Compact directional terahertz sources would be a boon to developing an underused part of the spectrum. Much work remains to be done in developing other components needed for terahertz technology. But QCLs are advancing and could become key components for future terahertz systems from astronomy to homeland security.
REFERENCES
1. J. Faist, F. Capasso, D.L. Sivco, C. Sirtori, A.L. Hutchinson, A.Y. Cho, Science, 264, 553 (1994).
2. R. Köhler et al., "Terahertz semiconductor-heterostructure laser," Nature, 417, 156-159 (2002).
3. B. Williams, "Terahertz quantum cascade lasers," Nature Photonics, 1, 517-525 (September 2007).
4. M.I. Amanti, M. Fischer, G. Scalari, M. Beck, J. Faist, "Low-divergence single-mode," (doi:10.1038/nphoton.2009.168).
5. N. Yu et al., Nature Mat., 9, 730-735 (September 2010; doi:10.1038/NMAT2822).
6. R.F. Curl et al., "Quantum cascade lasers in chemical physics," Chem. Phys. Lett., 487, 1-18 (February 2010).
Editor's note: Quantum cascade lasers will be discussed in detail at a panel discussion in the Technology Forum at the 2011 Lasers & Photonics Marketplace Seminar. See www.marketplaceseminar.com.
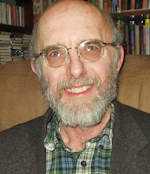
Jeff Hecht | Contributing Editor
Jeff Hecht is a regular contributing editor to Laser Focus World and has been covering the laser industry for 35 years. A prolific book author, Jeff's published works include “Understanding Fiber Optics,” “Understanding Lasers,” “The Laser Guidebook,” and “Beam Weapons: The Next Arms Race.” He also has written books on the histories of lasers and fiber optics, including “City of Light: The Story of Fiber Optics,” and “Beam: The Race to Make the Laser.” Find out more at jeffhecht.com.