OPTICAL SENSORS: Plasmonic antenna on QCL facet creates chip-scale molecular sensor
Because many biological molecules such as proteins and pharmaceuticals have a natural resonance in the mid-infrared (IR; 2 to 30 μm) portion of the electromagnetic spectrum, molecular biosensors that use mid-IR sources are highly desirable. Unfortunately, there is a weak light-matter interaction between mid-IR sources and biological molecules since the lasing wavelength is so much longer than the size of the molecules to be probed.
To overcome this hurdle, researchers in the Bio-inspired Sensors and Optoelectronics Lab (BISOL; www.bisol.northwestern.edu), led by professor Hooman Mohseni in the department of Electrical Engineering and Computer Science at Northwestern University (Evanston, IL), are integrating optical nano-antennas with quantum cascade lasers (QCLs) that focus the light down to the nanometer scale and strongly enhance the near-field intensity.1
A hybrid approach
Many plasmonic nano-antenna designs use all metallic materials; however, the hybrid metal-dielectric-metal (MDM) plasmonic nano-antenna from Northwestern confines the mode within a nanometric spot size of approximately 500 nm–a 4X near-field intensity enhancement compared to all-metal designs.
To optimize the MDM nano-antenna design, finite-difference time-domain (FDTD) modeling software was used to model the peak intensity enhancement of the near field versus the length of the nanorod-based antenna coupled to the front facet of a QCL. To fabricate the structure, the surface of an indium aluminum arsenide/indium gallium arsenide-based QCL was first coated with a gold/silicon dioxide/gold MDM sandwich (thickness 75/30/75 nm, respectively). Focused-ion-beam milling was then used to create the nanorod structure on the QCL front facet; nanorod dimensions are roughly 2.0 μm × 200 nm.
To observe the near-field images and test the topography of the patterned QCL, a mid-IR near-field scanning optical microscope setup was used (see figure). In the experiment, the apex of a sharp platinum-coated atomic-force-microscope (AFM) tip scans the surface of the QCL (operating in pulsed mode with a 1% duty cycle–100 ns pulsewidth at 100 kHz) and scatters the electromagnetic field coming from the antenna structure. The scattered signal travels back through the laser cavity, is collimated, then focused by an objective lens to a mercury-cadmium telluride (MCT) detector. Lock-in techniques remove the noise from the AFM tip and the resulting signal yields an image of the near-field intensity pattern of the fabricated nano-antenna.
For the nano-antenna QC laser structure, the full-width half-maximum (FWHM) of the center spot was approximately 450 nm. With a laser wavelength of approximately 6 μm, two bright hot spots are observed within the FWHM due to plasmon hybridization.
In a hybrid design like MDM, there are two surface-plasmon waves supported at the two interfaces between the metal and the dielectric. Unlike the single metal design, interaction between these multiple surface plasmons splits the degeneracy and produces symmetric and antisymmetric modes. In an MDM-coupled nano-antenna structure with greater geometrical complexity, there is hybridization between the symmetric and antisymmetric modes at certain frequencies, which leads to the two bright spots observed in the experiment.
A biosensor roadmap
The localized field enhancement helps increase the light-absorption efficiency of the molecule if it has a vibrational energy that matches the lasing frequency. The challenge for the plasmonic QCL integrated nano-antenna-based biosensor is how to position a biomolecule at the specific position where the near field gets localized. The researchers propose dip-pen nanolithography as a means to deliver a biomolecule to the mid-IR laser's hot spot.
"This result provides the first step towards developing a fully integrated, self-enclosed lab-on-a-chip-scale molecular detector, which is very important for delivering point-of-care information to EMTs and doctors," says Mohseni.
"A sensor based on a molecule's vibrational fingerprint is inherently more sensitive than conventional techniques such as Raman, and we believe our technique produces an extremely compact realization of a mid-IR biosensor," adds Dibyendu Dey, graduate student at BISOL.
REFERENCE
1. D. Dey et al., Opt. Lett., 35, 16, 2783-85 (2010).
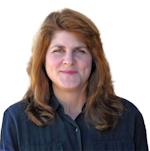
Gail Overton | Senior Editor (2004-2020)
Gail has more than 30 years of engineering, marketing, product management, and editorial experience in the photonics and optical communications industry. Before joining the staff at Laser Focus World in 2004, she held many product management and product marketing roles in the fiber-optics industry, most notably at Hughes (El Segundo, CA), GTE Labs (Waltham, MA), Corning (Corning, NY), Photon Kinetics (Beaverton, OR), and Newport Corporation (Irvine, CA). During her marketing career, Gail published articles in WDM Solutions and Sensors magazine and traveled internationally to conduct product and sales training. Gail received her BS degree in physics, with an emphasis in optics, from San Diego State University in San Diego, CA in May 1986.