Diode Lasers: Research gives high-power diode lasers new capabilities
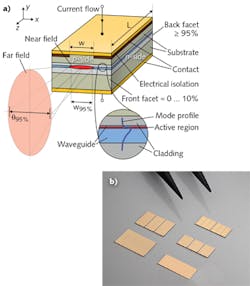
Many major laser applications in industry and academia are requiring ever-better diode-laser performance. Increasingly complex studies of the device physics are being used to guide developments in epitaxial layer design and device technology, and the resulting improved diode lasers are delivering the higher efficiency, brilliance, and output power that is urgently needed now and in the coming years.
Diode lasers (see Fig. 1) have become the silent heroes of industrial laser technology. They do the tough job of pumping the fiber and disk lasers that have conquered the multibillion-euro (and multibillion-dollar) market of cutting and welding—soon, they will challenge even these systems with direct-diode applications. On the academic side, new ultrashort-pulse high-energy lasers with high repetition rates are projected to open up new horizons in particle acceleration. These lasers will also be diode-pumped.
All these developments and more require better diode lasers, and with improved performance and reduced price per watt. While this sounds challenging, the established market for high-power diode lasers is growing fast and diversifying rapidly enough to encourage worldwide development efforts.
One of the hot spots for such R&D activities is Berlin Adlershof, with the Ferdinand-Braun-Institut, Leibniz-Institut für Höchstfrequenztechnik (FBH) and other academic institutions located on the same campus in Berlin, Germany. Beside several FBH spinoffs, larger companies such as the Trumpf Group, Jenoptik, II-VI, and Corning have subsidiaries within walking distance of the institute, where they work together on future developments in high-power diode lasers.
How to improve diode laser technology
Diode lasers are well known as the world’s most efficient light source, with records of more than 65% wall-plug efficiency at an emitted power of 12 to 15 W from a 100 µm aperture. High-power laser diodes are typically grown using metalorganic vapor-phase epitaxy (MOVPE) on gallium arsenide (GaAs) wafers with typical emission wavelengths between 700 and 1100 nm.
Although the discovery of diode lasers dates back to the early 1960s, diode laser parameters are still rapidly improving—specifically efficiency, peak power, brilliance, and the range of emission spectra. The research team at FBH is playing a key role in advancing this field. Their research in device physics and material technology helps clarify and address performance limits, and design development based on this information maximizes performance of commercially important devices—for example, better pumps to enable higher performance in industrial disk or fiber laser systems. The FBH technology also makes novel devices and modules possible and enables new kinds of applications. Inventions are protected by a broad patent portfolio. Example performance records include the first 1 cm diode laser bars with 1.5–2 kW output power and bars with >60% conversion efficiency at 1 kW.
Results of the work at FBH are regularly presented at numerous conferences. A plenary talk on the latest progress and prospects in this exciting and industrially critical field will be given by Günther Tränkle, director of the FBH, at SPIE Photonics West 2019 (San Francisco, CA; February 2-7).
Triple asymmetric layer designs
In the best modern high-power diode lasers, peak power and efficiency are not limited by failure, but instead by fundamental loss mechanisms. A deep understanding of the physics within the diode and the device technology is therefore a prerequisite for targeting further improvements.
As an example, based on the results of many years of device research into limits to power and efficiency, the FBH team recently developed the concept of a triple asymmetrical epitaxial layer design. Such a design precisely manipulates the optical field and leads to both higher efficiency and higher output powers.1 The first asymmetry is within the semiconductor material of the diode structure: The p-layer is made as thin as possible, which reduces electrical resistance and optical losses. This is combined with a second asymmetry in the design of the cladding and waveguide inside the diode. The clad/guide asymmetry helps to couple unwanted optical modes into the substrate, preventing them from lasing. Finally, a third asymmetry in the graded profile of the refractive index for the layers on either side of the quantum well is introduced, allowing for fine-tuning of the optical field.
A shift of the optical field towards the quantum well increases optical gain without the need to adjust the p-side, which can remain thin for low loss. For the first time, this allows the simultaneous realization of low resistance, low optical loss, low power saturation, and a low threshold current. With this structure, an efficiency record of 66% at 10 W output for continuous-wave (CW) output (940 nm, 25°C) was achieved (69% peak efficiency), 1.05X higher than comparable symmetric designs (see Fig. 2).Limits to efficiency also lie in the lateral structure, as significant levels of electrical current are lost on either side of the electrical stripe, even in broad-area lasers. A novel current blocking technology eliminates this lost current and is fabricated using a two-step in situ-etched MOVPE process. Corresponding prototype buried mesa lasers operated at a 5% higher efficiency (see Fig. 2). Combining both triple asymmetrical designs and lateral current blocking techniques is anticipated to further increase efficiency.
Efficient kilowatt laser bars
These sophisticated epitaxial designs, when fabricated into diode lasers using high-quality low-defect device technology, can be exploited for higher performance in applications. For example, 1 cm laser bars are in wide industrial use, directly and as pump sources, and increased power directly lowers cost per watt, reduces system size, and can also improve performance (via higher-brightness pumping). FBH research has helped address this challenge, enabling a two- to fourfold increase in the peak output power of diode laser bars over the last 10 years (see Fig. 3) in quasi-continuous-wave (QCW) mode for pumping of pulsed solid-state laser systems.In parallel, efficiency has been increased at 1 kW per bar, from approximately 35% to 63%. Currently, Trumpf and the FBH are collaborating closely, demonstrating kilowatt output in CW mode at the highest reported operating temperature of 298 K, and working to continuously increase efficiency and beam quality. Trumpf will report the latest progress at SPIE Photonics West 2019.
Monolithically wavelength-stabilized diode lasers
For applications such as solid-state laser pumping and sensing, diode lasers with a narrow and stable spectrum are needed. From the viewpoint of production costs, monolithically integrated gratings are particularly attractive technology for narrowing the spectrum—this approach eliminates the need for external feedback, which leads to lower lifetimes. As a result, FBH scientists have intensively studied design and technology for monolithic grating stabilization for many years, seeking to make this key technology ready for market application.Ultrabrilliant beam combining
Industrial systems combine the emission of many diode lasers into a single beam to reach the intensity needed to cut metals such as steel. Current systems use fiber or disk lasers to collect the energy from the diode lasers. Direct-diode laser systems have the potential to be a highly efficient, compact, and reliable competitor to these established solutions—therefore, intensive research is aiming to make this possible.
One important technique to increase the optical intensity from direct-diode sources is wavelength beam combining (WBC), in which light from many diode lasers are brought together into a single beam using, for example, an external grating. In this way, very high combined powers are produced, at the cost of broadening the spectrum. The FBH develops highly efficient ultra-intense arrays of diode lasers specifically for this application. Examples include high-power arrays of single-mode lasers that are tailored for spectral stabilization with an external grating and then wavelength-beam-combined (developed in a collaboration with Trumpf).
If the grating is brought inside the diode laser, the system can potentially be much simpler and more compact—a concept studied in detail in the EU-funded project BRIDLE (developed in a collaboration with DILAS and the Fraunhofer Institute for Laser Technology [Fraunhofer ILT; Aachen, Germany]). An array of five custom diode lasers with monolithically integrated gratings was spectrally combined and coupled into a low-mode optical fiber with 35 µm diameter and a numerical aperture (NA) of 0.2, with a total power from the fiber of 26 W in a single polarization—more than twice the intensity of commercially available unpolarized modules with 105-µm-diameter fibers.
A more advanced approach is coherent beam combination (CBC). Here, the output from one distributed feedback (DFB) seed laser is split into several beams whose powers are boosted via parallel semiconductor amplifier stages. Careful regulation of the amplifier currents hold their output perfectly in phase so that the array of amplifiers acts like a single laser, with a diffraction-limited beam and narrow spectrum. The FBH is studying how best to realize such systems (in a collaboration with the Laboratoire Charles Fabry (Paris, France) and the Technical University of Denmark (Kongens Lyngby, Denmark), and recent studies showed that CBC not only increases power but also improves beam quality, enabling generation of more than 2 W of frequency-converted green light—the highest reported level from a direct diode source.
World’s first joule-class mid-infrared laser light source
Advances in high-power diode lasers also open up new applications in solid-state lasers. In August 2018, partners from the German regions of Berlin and Jena joined forces in a new BMBF (German ministry for education and research) project, coordinated by the Berlin-Brandenburg Competence Network for Optical Technologies (OpTecBB). With 1.5 million euros, the team will develop the world’s first high-energy class mid-infrared laser (HECMIR), targeting pulse energies of more than 1 J at a wavelength of 1.9 µm. These pulse energies are made possible by highly intense, long-pulse diode laser pump sources, which will be developed and realized for the HECMIR by the FBH, exploiting novel diode laser designs and innovative high duty-cycle stacked array technology.
The short-pulsed MIR sources from HECMIR could enable particle (such as proton) beams from a tabletop device at an energy level that nowadays require basketball-court-sized accelerators. These particle beams would be an ideal noninvasive medical treatment for tumor removal. MIR sources are also projected to play a key role in future very-high-energy pulsed research lasers. A new generation of ultrashort-pulsed high-energy lasers is being installed worldwide, and there is demand for even higher performance. This will only be possible with advanced diode pumping, in both conventional and MIR wavelength bands, as is currently being studied in the EuPRAXIA consortium.2
REFERENCES
1. P. Crump, “Lasers: excelling with extreme asymmetry,” Compound Semiconductor, 24, 6 (Sep. 2018).
2. See www.eupraxia-project.eu.
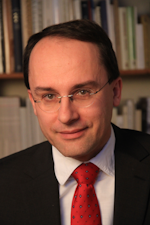
Andreas Thoss | Contributing Editor, Germany
Andreas Thoss is the Managing Director of THOSS Media (Berlin) and has many years of experience in photonics-related research, publishing, marketing, and public relations. He worked with John Wiley & Sons until 2010, when he founded THOSS Media. In 2012, he founded the scientific journal Advanced Optical Technologies. His university research focused on ultrashort and ultra-intense laser pulses, and he holds several patents.
Paul Crump | Head, High-power Diode Lasers Laboratory at the Ferdinand-Braun-Institut (FBH)
Paul Crump is the head of the high-power diode lasers laboratory at the Ferdinand-Braun-Institut (FBH; Berlin, Germany).