Broadly tunable lasers would be a boon to both developers and users of wavelength-division multiplexed (WDM) fiberoptic systems. No one wants to maintain an inventory of dozens of different laser transmitters, one for each optical channel. Stocking spares for each channel at every telephone-switching center with a WDM system would create logistical nightmares for telecommunications carriers.
The huge potential demand has created a quest for the ideal tunable laser, able to generate constant output power across a broad range of wavelengths so it could serve as a near-universal replacement. Its wavelengths should be adjustable on-site and—ideally—from remote operations centers. The output wavelength and power should be stable in an operating environment, and the costs should be reasonable. It's a tall order, but considerable progress has been made. Several approaches are under development, and several types are commercially available. So far most applications have involved instrumentation, but some companies have targeted the large market for WDM transmitters. Watch for interesting new results in the technical sessions and on the show floor at this month's Optical Fiber Communications Conference (Anaheim, CA).
Types of tuning
The two key building blocks for a tunable laser are an active medium with laser gain across an adequate range of wavelengths and a laser resonator that can limit oscillation to a narrow band of wavelengths. Both semiconductor-diode lasers and erbium-doped fiber lasers offer broad gain bandwidths, but so far most development of tunable WDM sources has focused on diode lasers.
Tuning mechanisms are diverse. One approach, which traces back to early tunable dye lasers, uses dispersive optical elements—typically diffraction gratings—in an external cavity to select one resonant wavelength. Another approach moves an external mirror back and forth to change the cavity length and thus the resonant wavelength. Several distinct approaches are based on changing the resonant wavelengths of distributed Bragg reflectors serving as cavity mirrors in semiconductor lasers. A distinct alternative is a monolithic device that selects one laser stripe from an array of several emitting at different wavelengths, with fine-tuning of individual lines for precise wavelength control.
External-cavity diode lasers
The external-cavity diode laser typically is an edge-emitting laser with an antireflection coating applied to one cleaved facet. This extends the cavity on that side of the laser to include a dispersive element and a mirror. The dispersive element spreads out a range of wavelengths, and adjusting it and the mirror select which wavelength is reflected back into the laser cavity. This feedback makes the laser oscillate at the selected wavelength.
In the simple Littrow cavity, a reflective diffraction grating serves as both mirror and dispersive element; tilting it back and forth selects the wavelength. The more complex Littman cavity is used in tunable diode lasers developed for instrumentation and fiberoptic applications (see Fig. 1). Pivoting the remote mirror around a specific point simultaneously changes the length of the cavity and the resonant wavelength fed back into the semiconductor gain medium. Light reflected from the grating at a glancing angle becomes the laser output. New Focus (Santa Clara, CA) uses this approach in its tunable diode lasers, and for 1550-nm sources claims a tuning range of 70 nm and bandwidth of 300 kHz. The company originally developed the lasers for instrumentation, and now is extending the technology to tunable sources for WDM systems.
MEMS-tunable VCSELs
Another class of tunable laser relies on a moving external mirror, but in this case it tunes the output wavelength of a vertical-cavity surface-emitting laser (VCSEL) by changing the cavity length. As described in last month's Optical Networking article (see Laser Focus World February 2000, p. 123), VCSELs have very short cavities, with widely separated longitudinal modes. This means that moving the cavity mirror a few micrometers may change the resonant wavelength a few percent, which is enough for WDM applications.
The mirrors are microelectromechanical systems (MEMS), formed by depositing additional semiconductor layers on a VCSEL. Etching away the intermediate layers leaves a flexible layer suspended above the VCSEL. Passing electrical signals through conductors fabricated on the semiconductor moves this flexible layer up and down, changing the length of the VCSEL cavity and the resonant wavelength. Bandwidth9 (Fremont, CA) is developing electrically pumped lasers based on this technology. CoreTek (Burlington, MA), which Nortel bought last year, has demonstrated optically pumped VCSELs with similar tuning mirrors.
Tuning DFB and DBR structures
Distributed feedback (DFB) and distributed Bragg-reflection (DBR) lasers were developed for their single-wavelength output. Because the wavelength is selected by the spacing and refractive index of the DFB or DBR grating, adjusting either could tune the laser. In practice it is easier to change the refractive index, which depends on both temperature and current density, allowing development of temperature- and current-tuned lasers. Tuning ranges typically are limited to about a couple of nanometers in single DFB lasers, in which the grating is in the laser's active area.
Tuning over ranges of more than 10 nm is possible in DBR lasers in which the grating is outside the laser zone (see Fig. 2). The DBR structure is electrically isolated from the active stripe, and changes in its operating temperature and/or in the current passing through it alter its refractive index, changing the wavelength it selectively reflects. In the simple example of Figure 2, the device includes only a single zone of distributed Bragg reflection, but structures with multiple zones have the highest tuning ranges. They also may contain a smaller phase region for fine tuning. A number of companies have developed tunable DBR lasers, including Lucent Technologies (Murray Hill, NJ), Alcatel Optronics (Nozay, France), and Multiplex Inc. (South Plainfield, NJ).Multistripe arrays
A fundamentally different approach to tunability is building monolithic arrays of multiple DFB or DBR laser stripes with different center wavelengths and limited tuning ranges, and switching among the stripes to give broader tuning range. Only one stripe operates at a time, selected by control circuits that may fine-tune its wavelength to match the optical channel requirements. Waveguides channel the output from all stripes into a coupler that combines them into a single output. The user doesn't know or care about the extra stripes; the monolithic device functions as a single laser, tunable across a range equal to the number of stripes times the tuning range of each one. With a dozen stripes, a DFB design tunable across only a 2-nm range per stripe could collectively span 24 nm.
Erbium-doped fiber lasers
Erbium-doped fiber lasers also have broad gain bandwidth. Tunable erbium-fiber lasers have been developed for instrumentation, and pumped erbium fibers without cavity mirrors can serve as broadband sources of amplified spontaneous emission for measurement applications. However, making tunable erbium-fiber lasers with the stable narrow-line output needed for WDM systems is difficult because the laser cavity normally contains at least a meter of fiber. David Payne, founder of Southampton Photonics and director of the Optoelectronics Research Center at the University of Southampton (Southampton, England), points out that longitudinal modes are only about 100 MHz apart, much closer than the modes in short-cavity diode lasers.
Payne says that arrays of fixed-wavelength fiber lasers offer a way to avoid the need for tunable replacement lasers. The trick is pumping with an array of diode lasers arranged so that their output is combined, then distributed among all the fibers, so a single laser failure would not disrupt any optical channel. Southampton Photonics is working on this approach, which Payne notes would avoid the difficulty of combining tunability with long-term wavelength stability.
Erbium-fiber lasers also can generate broadband continuum emission in ultrashort pulses. Fiber dispersion can chirp and stretch those pulses so their output wavelength changes during each pulse. A properly synchronized modulator can slice each multiwavelength pulse into a series of pulses at many distinct wavelengths, generating many optical channels from a single source. So far the technique has been demonstrated only in the laboratory, but it has interesting potential.
Seeking practical devices
The huge potential market is driving intense development, and new approaches such as quantum dots continue to emerge, but some important issues remain. Output power is a concern because some laser types have only low output, or have output that varies significantly with wavelength. Long-term stability of both wavelength and output power is a concern for any new device, as is its ability to withstand realistic operating environments. A final challenge is manufacturing devices at reasonable prices and in adequate quantity. It may be a few years before we know the winners of the development race.
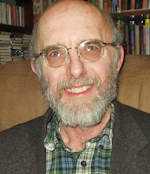
Jeff Hecht | Contributing Editor
Jeff Hecht is a regular contributing editor to Laser Focus World and has been covering the laser industry for 35 years. A prolific book author, Jeff's published works include “Understanding Fiber Optics,” “Understanding Lasers,” “The Laser Guidebook,” and “Beam Weapons: The Next Arms Race.” He also has written books on the histories of lasers and fiber optics, including “City of Light: The Story of Fiber Optics,” and “Beam: The Race to Make the Laser.” Find out more at jeffhecht.com.