PHOTONIC FRONTIERS: LASER FUSION: Will NIF number nuclear fusion among its accomplishments?
In March 2012, the giant National Ignition Facility delivered a record pulse containing 1.8 MJ of ultraviolet (UV) light to a target at the Lawrence Livermore National Laboratory (Livermore, CA). The culmination of years of effort, which met the design goal for the world's biggest laser, exhibits an impressive achievement in overcoming tough technical problems such as laser damage (see "Overcoming optical damage").
By the end of 2012, the laser shown in Fig. 1 had fired more than 1100 shots—an average of one per day since completion—and was being used to produce extreme conditions to study the frontiers of physics and simulation of nuclear weapons.
But the exceptional performance that exceeded design specifications failed to ignite nuclear fusion in sophisticated cryogenic targets of deuterium and tritium, the goal of the three-year National Ignition Campaign, which ended Sept. 30, 2012. Theory and computer models developed over many years of lower-energy experiments predicted that 1.8 MJ pulses should ignite nuclear fusion, producing more energy from fusion reactions than the laser pulse delivered to the target. But that theory failed the acid test of experiment.
Neutron yield, which measures the extent of fusion reactions, increased during the NIF experiments, but the best results were a factor of 3 to 10 below ignition. Compression of the nuclear fuel was only half that required for ignition, the National Nuclear Security Administration reported.1 Now researchers and government officials are investigating what went wrong with the physics and how to reach ignition.
A half-century quest for fusion
Laser fusion has been alluring since Livermore physicist John Nuckolls conceived the idea half a century ago. Early calculations suggested that a kilojoule pulse should heat and compress a deuterium-tritium target enough to produce a kilojoule of fusion energy.2 Experiments in the 1970s produced fusion neutrons but showed the need for much more laser energy at shorter wavelengths.
When Livermore planners designed the 10-beam Nova laser, they hoped it would point the way to ignition at 1 MJ, but experiments after it was completed in 1985 suggested the threshold was closer to 10 MJ, an energy that seemed out of reach in the 1980s. However, by 1992 further work and new target designs suggested UV pulses of 1 to 2 MJ could trigger ignition, and Nuckolls—then head of Livermore—proposed building NIF.3
The timing was fortunate. The incoming Clinton Administration wanted to stop nuclear testing, and laser fusion offered a way to simulate nuclear-weapon physics without underground tests. NIF became a centerpiece of the Department of Energy's Stockpile Stewardship Program to maintain the US nuclear arsenal under the Comprehensive Nuclear-Test-Ban Treaty.
Projected in 1994 to cost about $1 billion and be completed by 2002, NIF hit serious technical and management problems in 1999. Livermore brought in Ed Moses to overhaul the project, which wound up costing $3.5 billion when it was declared operational in March 2009.4 It initially delivered 1.1 MJ pulses, and reached the 1.8 MJ goal in March 2012 after careful adjustments.
Early on, Livermore was optimistic about ignition. In early 2010, they reported the first symmetrical explosions and talked of ignition at 1.2 to 1.3 MJ.4 They proposed building a follow-on pure-fusion reactor powered by diode-pumped solid-state lasers (DPSS) called Laser Inertial Fusion Energy.5 They hoped LIFE could produce commercial power in the 2020s, before ITER, the international magnetic-confinement fusion reactor being built in France.
Implosion quality improved substantially during a series of 37 cryogenic target experiments aimed at ignition. NIF also performed other tests for stockpile stewardship and fundamental physics research. Current experiments are producing conditions like those in the core of Jupiter, and, says NIF director Ed Moses, "We're about two-thirds of the way to the center of the Sun." Moses boasts that NIF pulses are repeatable to within 2%, and that only the complexity of experimental hardware limits the laser to one shot per day.
But Congress wanted ignition, and required NNSA to report by December 2012 what had gone wrong and how to fix it if NIF did not ignite a fusion plasma by the end of fiscal 2012.
Approaches to inertial confinement
The NNSA report stresses that NIF worked precisely and reliably, meeting the design specification that had been supposed to reach ignition. That meant the failure was in understanding the physics well enough to accurately model the process of inertial confinement fusion.
Laser fusion is often described as imploding a fusion target with laser pulses that heat the fusion fuel and pushing it inward to produce a hot, dense, short-lived plasma. However, NIF uses a more complex process called indirect drive fusion, in which its 192 beams illuminate the interior of a small metal cylinder called a "hohlraum" that contains the frozen hydrogen target, as shown in Fig. 2. The laser pulse ionizes the metal, producing a powerful burst of x-rays that heat and compress the target. That technique is supposed to heat and compress the target more efficiently and more uniformly than directly illuminating the target with laser light. Uniform compression is particularly important for plasma confinement. Another advantage of indirect drive fusion is that it mimics the physics of a thermonuclear bomb, where the x-rays from a fission explosion heat and compress the bomb's fusion stage. The strong connection with weapon physics has been the DOE's main reason for supporting laser fusion research since its early days at Livermore; fusion energy was a secondary interest.NIF shots are needed to verify computer models developed on theory and earlier experiments. The models extrapolated results from tests with the 30 kJ Nova to the 60-times-higher UV energies that NIF deposits on the hohlraum, and predicted that 1.8 MJ should ignite the deuterium-tritium fuel pellet. Data from heavily instrumented NIF experiments showed that the computer models were overly optimistic. Measured target compression reached only about half the forecast level, with measured neutron yields a factor of 3 to 10—too low for ignition. Estimates of the shortfalls are only approximate because ignition, like a laser approaching threshold, starts slowly but later goes very fast.
The models appear to be missing crucial physical processes that reduce fusion yield, but it's not yet clear what those processes are. The NNSA reports lists a series of discrepancies including "opacities and equations-of-state (EOS) disagreeing with models; low-mode asymmetry being larger than expected; capsule drive being lower than expected as a result of inadequate models of hohlraum and laser plasma interactions; and a hydrodynamic mix cliff occurring at lower in-flight aspect ratios than predicted by present models."1
Moving beyond the ignition campaign
This year NIF will devote half of its time to stockpile stewardship and high-energy-density physics, 40% to ignition research, and 10% to fundamental physics. Moses reports plenty of interest, with qualified requests for 500 shots this fiscal year, about twice NIF's capacity.
Through 2015, NNSA is shifting the emphasis of its ignition research program "to illuminate the physics and to improve models and codes used in the ICF program until agreement with experimental data is achieved." The project will use NIF, the OMEGA laser at the University of Rochester (Rochester, NY) and the Z Pulsed Power Facility at Sandia National Laboratories (Albuquerque, NM) to learn what is needed for ignition and how well NIF can meet those requirements.
One series of experiments will focus on resolving specific inertial-fusion issues such as measuring instabilities, improving equations of state, and refining algorithms used in computer codes. Integrated experiments, like those done during the ignition campaign, will probe issues such as mixing and asymmetry of implosions under different conditions. Additional tests will examine the physics of x-ray-driven implosions. Current plans for 2013 call for 25% of Z-machine time and 35% of Omega shots as well as 40% of NIF shots to go to ignition studies. All three will also be used for stockpile stewardship and physics research, with NIF's high-energy offering unique capabilities.
Other types of inertial fusion
NNSA also wants to study alternate approaches to inertial confinement fusion.
Rochester and the Naval Research Laboratory (Washington, DC) have long pursued direct drive, with the beams directly illuminating the target. "Simulations predict that direct-drive can couple 6 to 9 times more energy to the capsule" than using the same laser energy for indirect-drive fusion, says Robert McCrory, director of the Laboratory for Laser Energetics at Rochester. "This extra energy provides direct drive with a larger design space than is available with indirect drive."
So far, most direct-drive experiments have sought to produce symmetric implosions by illuminating spherical targets uniformly across their entire surface. Rochester's 60-beam OMEGA laser was designed to deliver a total of 40 kJ for that purpose. The optics of the much larger NIF were designed to illuminate hohlraums from the top and bottom, and would require costly and time-consuming modification for symmetric direct-drive tests. Instead, Rochester is developing an alternative polar direct-drive approach, in which beams arrive from above and below the target as shown in Fig. 3. Increasing the power of beams incident at oblique angles near the equator of the target helps produce more symmetric implosions. McCrory says modifying NIF for polar direct drive should cost only about $40 to $50 million over four years and would have only minimal impact on NIF operation. Polar direct drive also may be usable in some high-energy-density experiments for stockpile stewardship.A second alternative is magnetically driven implosions now used to produce bright x-rays for radiation effects research. They use pulsed power machines such as Sandia's Z machine to heat and compress plasmas by collapsing magnetic fields. NNSA is investigating them for stockpile stewardship and inertial confinement fusion.
Controversy over NIF
NIF has some harsh critics. It was "built for largely political reasons," at a time when Livermore needed new programs and the Clinton Administration needed support for the comprehensive test ban treaty, says long-time critic Christopher Paine of the National Resources Defense Council (Washington, DC). "It wasn't critical to stockpile stewardship and the lab directors knew it." Livermore "extrapolated the physics too far and without sufficient basis, and they believed too much in their computer predictions," wrote Stephen Bodner, retired head of laser fusion at the Naval Research Lab in a critique dated Nov. 11, 2012.
Others thought Livermore's proposal for a laser fusion power plant was premature. "There remain critical scientific and engineering challenges associated with establishing the technical basis for an inertial fusion energy demonstration plant," warned a National Research Council review panel in a March 2012 interim report. (A full report is due out in January 2013, too late to be covered here.)
But the most crucial critics NIF will face this year are budget hawks in Congress. NIF has funds for fiscal 2013, but Congress has been getting impatient. The House and Senate Appropriations Committee and the House Armed Services Committee all worried about NIF not reaching ignition in discussing the fiscal 2012 budget. That could leave NIF vulnerable when the committees write the fiscal 2014 budget.
Outlook
The good news is that Livermore has created a unique laser facility, with unmatched capabilities for high-energy-density physics with applications from basic research to controlled nuclear fusion and nuclear weapons. It's huge, complex, tremendously powerful, and working very well. NIF has fired up to four shots in a day but usually is limited to one per day by the complex instrumentation needed for measurements. With $3.5 billion invested in building NIF, and users lined up for shots, it would seem logical to follow through with planned research. A performance upgrade may even be possible.
The bad news is that NIF operations are expensive. NNSA spent $475 million on the inertial confinement fusion ignition and high-yield campaign in fiscal 2012, and is slated to receive nearly as much in fiscal 2013. Politicians under pressure to slash spending can make penny-wise but pound-foolish decisions. Let's hope they recognize NIF as an asset to be used productively.
REFERENCES
1. National Nuclear Security Administration's Path Forward to Achieving Ignition in the Inertial Confinement Fusion Program, Report to Congress (December 2012); http://fire.pppl.gov/NIF_Path_Forward_Rpt_120712.pdf.
2. J. Nuckolls et al., "Laser compression of matter to super-high densities: thermonuclear (CTR) applications," Nature, 239, 139–142 (Sept. 15, 1972).
3. J. Nuckolls, "Contributions to the genesis and progress of ICF," in Inertial Confinement Nuclear Fusion: A historical Approach by its Pioneers, G. Velarde and N. Santamaría eds., Foxwell & Davies (UK) Ltd. (2007).
4. J. Hecht, "Photonic Frontiers: The National Ignition Facility: NIF is up and running at last," Laser Focus World, 45, 11 (Nov. 2009); http://bit.ly/ZYaBbS.
5. Interim Report-Status of the Study "An Assessment of the Prospects for Inertial Fusion Energy," National Academies Press (March 2012); http://www.nap.edu/catalog.php?record_id=13371.
Overcoming optical damage
Among the most impressive achievements in building and operating the National Ignition Facility is successfully controlling the optical damage that has long limited the performance of high-power solid-state lasers.
Damage-prone platinum inclusions in the first production runs of laser glass for Livermore's earlier Nova laser required replacement before that system could reach its full power level. NIF, operating at much higher powers, posed major challenges in preventing damage to laser glass, coatings, optics, and harmonic-generation crystals. But NIF reliably delivered pulses with peak power of 500 TW, despite operating above the damage threshold for the final optics delivering the 351 nm third harmonic to the target.
That achievement required understanding damage processes, identifying precursors to damage, and improving glass processing and polishing techniques. Livermore also developed tools for controlling incipient damage. A Final Optics Damage Inspection system uses a telescope in the target chamber that can look back through the optical system to check for damage in all 192 beams in a four-hour period. The system tracks changes in small damage zones, and when the affected zone reaches a risky size, it controls optical switches that block light from reaching that zone. That stops spread of the optical damage without significantly reducing power. When slightly damaged zones become risky, the optics can be swapped for new or refurbished components.
"Without this ingenious strategy developed by the NIF staff to minimize optical damage in the laser, it would be impossible to operate the NIF laser at its specified wavelength, energy and power," wrote a review panel headed by Robert Byer of Stanford University (Stanford, CA). The panel concluded that the success of that strategy and other improvements opens "a credible path for upgrading the NIF laser to 3-MJ, 700 TW performance."
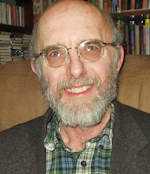
Jeff Hecht | Contributing Editor
Jeff Hecht is a regular contributing editor to Laser Focus World and has been covering the laser industry for 35 years. A prolific book author, Jeff's published works include “Understanding Fiber Optics,” “Understanding Lasers,” “The Laser Guidebook,” and “Beam Weapons: The Next Arms Race.” He also has written books on the histories of lasers and fiber optics, including “City of Light: The Story of Fiber Optics,” and “Beam: The Race to Make the Laser.” Find out more at jeffhecht.com.