Holographic techniques ‘key’ to nanofabrication deep within a silicon wafer
In a breakthrough for photonics, photovoltaics, and electronics, researchers at Bilkent University in Turkey came up with a new way to fabricate nanostructures deep inside silicon wafers. Traditionally, it’s been limited to surface-level nanofabrication via lithography.
But there’s plenty of room at the bottom of silicon wafers, the unused bulk, and it’s what Onur Tokel, a physics professor, and his group opted to exploit.
Their work opens the door to fabricating at the nanoscale directly within silicon, which should make it easier to usher in advanced photonics and possibly even achieve the dream of complete three-dimensional (3D) nanofabrication within silicon (see video).
Holographic projection
Tokel’s group zeroed in on the challenges of complex optical elements within silicon wafers and the inherent diffraction limit of the laser light.
“In linear optics, the minimum achievable feature size is given by the diffraction limit, which is at best half the wavelength of the laser light,” says Tokel. “This limitation is relaxed if we exploit nonlinear effects. The nonlinear effects within the bulk of the wafer are closely linked to the spatial and temporal distribution of the laser pulses. To exert control on these, the team used advanced holographic projection techniques.”
By using a spatial light modulator (SLM), they can create laser pulses with intensity patterns that correspond to a Bessel function. Bessel beams are special because they’re a nondiffracting form of light propagation and, in this case, it enables precise energy localization. And this leads to high temperature and pressure values that make it possible to modify the silicon at a small volume.
“We discovered that holographic techniques can shape a laser beam into nondiffracting Bessel beams, rather than traditional Gaussian beams, to achieve nanofabrication deep within a silicon wafer,” explains Tokel. A nondiffracting beam overcomes scattering effects that previously prevented precise energy deposition, and instead induces extremely small, localized voids within the wafer.
Using laser pulses at a wavelength—1550 nm—where the wafer is transparent means pulses can penetrate the bulk silicon without altering its surface. “This approach triggers various nonlinear effects and leads to localized energy deposition, which enables material modification at the nanoscale—as well as diverse architectural possibilities,” Tokel says.
The researchers follow this step with an emergent seeding effect, in which preformed subsurface nanovoids create strong field enhancement around their immediate area, to achieve feature sizes down to 100 nm. It’s based on localizing the energy of the laser pulse within silicon to an extremely tiny volume (comparable to that of nanoparticles), then exploiting emergent field enhancement effects analogous to plasmonics.
They demonstrated large-area volumetric nanostructuring with beyond-diffraction-limit features to enable proof-of-concept buried nanophotonic elements. It opens a pathway to nanoscale systems with unique architectures.
Tokel believes the emerging design freedom within silicon—beyond-diffraction-limit features and multidimensional control—will find numerous applications in electronics and photonics, such as metamaterials, metasurfaces, photonic crystals, information processing applications, and even 3D-integrated electronic-photonic systems.
One of the coolest aspects of this work is that the resulting field enhancement, once established, sustains itself through the seeding mechanism. The use of laser polarization provides further control of the nanostructures’ alignment and symmetry to enable creating diverse nanoarrays with high precision.
No masks required
A significant advantage of the group’s approach is that it’s a direct-laser writing method, which means it eliminates the need for any masks or the multiple fabrication steps typically required for conventional nanofabrication.
“In this sense, the complexity is transferred to the hologram, which spatially modulates the laser beam,” says Tokel. “Since we use a spatial light modulator, the hologram can be changed as needed—increasing the dynamic range of fabrication.”
Polarized direction
One surprising aspect of the group’s work was discovering that the lithographic structures exhibit polarization direction. It relates to the existence of nanoscale voids within laser-modified sections, akin to plasmonic hotspots but positioned inside the wafer. “It’s exciting because if we can control these in three dimensions, it could lead to significant applications,” says Tokel. “Advanced control could enable unique 3D architectures, which, in turn, can be converted into novel optical elements. We can imagine exploiting the depth degree of freedom for optics at the nanoscale and directly within the bulk of the wafer.”
The group is already creating multilevel volume gratings via this approach. “These types of buried optical elements can, in principle, be combined with surface-level devices—and it enables a new fabrication paradigm we broadly call ‘in-chip,’” Tokel says.
One intriguing future direction is integration. “We’ve demonstrated multilevel nanofabrication in silicon, which enabled volume Bragg gratings,” Tokel says. “This could, in principle, be combined with surface fabrication, with silicon photonics, or other devices. We can tackle the challenges in light coupling challenges to silicon photonics or imagine multifunctional, multilevel devices.”
More work needed
Tokel emphasizes their work enables the initial steps of controlled bulk nanofabrication, but there is significantly more work to be performed for diverse applications.
One big challenge ahead is to enhance the architectural control within silicon’s bulk. “While we can fabricate nanoplanes or nanolines, creating nanoscale voxels (a 3D counterpart to a pixel) is still beyond our reach,” Tokel points out.
Another challenge is to achieve high-optical index contrast with respect to the unmodified crystal matrix, which is essential for developing 3D-integrated optics, advanced waveguides, photonic crystals, or meta-optics applications inside semiconductors. “Potential integration opportunities from such elements are vast,” Tokel says. “There’s plenty of space at the bottom of the wafer, but more work remains to be done.”
In-chip applications ahead
The group now plans to explore the fundamental limits of lithography, which can be translated into advanced in-chip nanoapplications in the future. It will, not surprisingly, require a deeper understanding of the physics of the system. “During laser-material interaction, for example, we observed that earlier structures facilitate the fabrication of subsequent structures,” says Tokel. “This phenomenon corresponds to a new seeding type effect in silicon that we’d like to exploit further.”
Beyond this, they plan to exploit the latest advances within the field of holography, such as 3D holographic projection capability. “Exerting further control over the laser-semiconductor interaction system is an exciting challenge that paves the way toward a variety of applications,” Tokel adds.
FURTHER READING
R. Asgari Sabet, A. Ishraq, A. Saltik, M. Bütün, and O. Tokel, Nat. Commun., 15, 5786 (2024); https://doi.org/10.1038/s41467-024-49303-z.
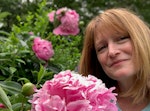
Sally Cole Johnson | Editor in Chief
Sally Cole Johnson, Laser Focus World’s editor in chief, is a science and technology journalist who specializes in physics and semiconductors. She wrote for the American Institute of Physics for more than 15 years, complexity for the Santa Fe Institute, and theoretical physics and neuroscience for the Kavli Foundation.