Metasurface Optics: Space-time optical metasurface produces nonreciprocal reflection
Conventionally, a light ray traveling in one direction through an optical system, when reversed in direction, would travel back to the point of origin of the original ray. However, optical devices can be made to defeat this convention. Optical components that support such unidirectional flow of light—for example, isolators and circulators—are currently typically based on the magneto-optic effect, making the devices bulky and difficult for integration into silicon photonics.
Other nonreciprocal devices in research or theoretical stages can rely on time-based spatial effects. For example, a bulk mirror consisting of two plates forming a thin, flat Fabry-Perot cavity with an acousto-optical material in between was proposed in 2018 that could, in theory, become a nonreciprocal mirror if acoustic waves with modulation frequencies of hundreds of megahertz were sent across the cavity in one direction.1
Scientists at the Nanophotonics and Optoelectronics Laboratory at Penn State (State College, PA) have now developed a type of optical metasurface that can impose phase modulation in both space and time the light reflected from it, leading to different paths for forward and backward light propagation (see figure).2 This device has experimentally shown nonreciprocal light propagation in free space at optical frequencies.
“This is the first optical metasurface with controllable ultrafast time-varying properties that is capable of breaking optical reciprocity without a bulky magnet,” says Xingjie Ni, a professor of electrical engineering at Penn State.
The device imposed time-based spatial effects on itself in a different manner from the Fabry-Perot-cavity-based device described above. The Penn State device is an ultrathin metasurface consisting of a silver back-reflector plate supporting block-shaped, amorphous-silicon nanoantennas that have a large nonlinear Kerr index and low loss at near-infrared (near-IR) wavelengths around 860 nm.
Traveling-wave index modulation
Heterodyne interference between two laser lines that are closely spaced in frequency is used to create efficient traveling-wave refractive-index modulation onto the nanoantennas, leading to ultrafast space-time phase modulation with an unprecedentedly large temporal modulation frequency of about 2.8 THz, breaking time-reversal symmetry for the device.
This dynamic modulation technique shows great flexibility in tuning both spatial and temporal modulation frequencies, according to the researchers. Completely asymmetric reflections in forward and backward light propagations were achieved experimentally with a wide bandwidth around 5.77 THz within a subwavelength interaction length of 150 nm; the experimental diffraction efficiency of the metasurface was 84%.
Light reflected by the space-time metasurface acquires a momentum shift induced by the spatial phase gradient as well as a frequency shift arisen from the temporal modulation. It exhibits asymmetric photonic conversions between forward and backward reflections. In addition, by exploiting unidirectional momentum transfer provided by the metasurface geometry, selective photonic conversions can be freely controlled by designing an undesired output state to lie in the forbidden (nonpropagative) region.
The approach, which can flexibly control light both in momentum and energy space, could lead to compact, integratable, magnet-free nonreciprocal devices for communications and other uses. The researchers note that 2D, rather than the experimentally shown 1D, spatiotemporal modulation could be created using three or more rather than two pump beams.
REFERENCES
1. R. Fleury, D. L. Sounas, and A. Alù, J. Opt. (2018); doi:10.1088/2040-8986/aaaa3e.
2. X. Guo et al., Sci. Appl. (2019); https://doi.org/10.1038/s41377-019-0225-z.
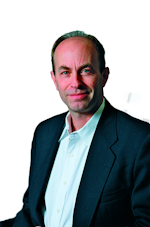
John Wallace | Senior Technical Editor (1998-2022)
John Wallace was with Laser Focus World for nearly 25 years, retiring in late June 2022. He obtained a bachelor's degree in mechanical engineering and physics at Rutgers University and a master's in optical engineering at the University of Rochester. Before becoming an editor, John worked as an engineer at RCA, Exxon, Eastman Kodak, and GCA Corporation.