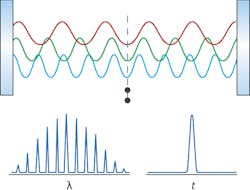
The short pulse durations and impressive peak powers of ultrafast lasers make them highly advantageous for a range of applications, including precise materials processing, micromachining, biomedical systems, communications, and nonlinear microscopy and imaging. Benefits for materials processing include better dimensional tolerances, a reduction of postprocessing steps required, and minimized damage to surrounding areas.1 In medical applications such as laser surgery, ultrafast laser sources result in decreased trauma and less anesthetics and sterilization required.2
Ultrafast lasers have an inherently wide wavelength bandwidth because of their short pulse duration, which leads to significant chromatic dispersion in optical media. Optical components with a negative group-delay dispersion (GDD), such as dispersive flat mirrors, are often used to balance the positive GDD of most optical media. However, a large number of reflections is required to compensate for high GDD, requiring the use of a cumbersome system of many flat mirrors. This issue can be solved through creating a multipass cell using one or more concave dispersive mirrors with through-holes. These multipass cells allow for maximum pulse compression and are more compact, cost-effective, and easier to align than previous approaches.
Dispersion compensation in ultrafast systems
Ultrafast lasers produce ultrashort laser pulses through mode-locking, which occurs when light waves are emitted coherently through in-phase superposition and contain a large quantity of modes (see Fig. 1).
Pulse compression is an essential part of ultrafast laser setups, as most high-energy ultrafast systems rely on this in a process called chirped-pulse amplification (CPA). By deliberately broadening an ultrafast pulse using a pulse stretcher, the peak power is substantially reduced, making it possible to amplify the pulse to even-greater energies without nonlinear pulse distortion or damaging of the gain medium or the surrounding optical elements. The amplified laser pulses are then recompressed into high-intensity ultrashort pulses (see Fig. 2).There are a number of different types of ultrafast pulse compressors that employ different types of optical components. Traditional pulse compressors use prism or diffraction grating pairs that are capable of compensating for large amounts of dispersion. However, GDD is usually tuned by changing the distance between the pair, which requires bulky mechanical setups in many laser systems and leads to high sensitivity to misalignment. Another disadvantage of prism and grating pairs is their low transmission or reflectivity, which leads to the loss of a substantial amount of the laser intensity.
Compressors based solely on mirrors with dispersive coatings are highly advantageous for pulse compression due to their alignment-free nature, low loss, and zero third-order dispersion. However, multiple reflections off many of these mirrors is usually necessary to achieve the GDD target needed, which makes the footprint of these compressors very large. The ability to reduce the size of all-mirror compressors would be highly advantageous to building compact ultrafast laser compressors. A successful compressor design requires an increase in the number of reflections between the dispersive mirrors to reach the target GDD while maintaining a small footprint for the overall setup. This strategy helps reduce the number of mirrors in the setup, which in turn lowers the cost of the compressor and leads to compact ultrafast lasers.
Multipass compression cells
Multipass cells have been commonly used in weak-trace absorption spectroscopy for decades to send a beam of light through a sample multiple times to look for weak absorption spectra. Sometimes a signal cannot be observed with one pass through a gas sample, but only by passing the beam through it multiple times. The total optical path is increased, which in turn leads to amplification of the absorption signal. A typical multipass cell consists of two concave mirrors or a concave and a flat mirror with through-holes that act as entrance and exit apertures for the cell (see Fig. 3). Such configurations provide a long optical path length ensured by the increased number of reflections in a simple and compact design that is very stable to small perturbations. When a beam is directed into such a cell, it gets reflected multiple times between the two mirrors, leading to a typical elliptical or circular pattern on the mirror surfaces.This multipass configuration can be applied to an all-mirror pulse compressor design by using highly dispersive mirrors, which allow for an increase of the number of reflections while limiting the number of mirrors used to only two. For example, a dispersive mirror coating with GDD of -1000 fs2 and reflectivity of >99.9% between 1010 and 1070 nm was applied to create a multipass compression cell. The GDD of the mirror (see Fig. 4) was measured using a white-light interferometer at 7° angle of incidence (AOI).
Adding dispersive mirrors to a multipass cell configuration is advantageous in building a compact, tunable, and alignment-free ultrafast compressor. A careful optical design of the system is necessary to obtain a high-quality beam. These types of compact compressors can be used in CPA laser systems or for dispersion compensation in any ultrafast laser setup requiring careful control and fine-tuning of the pulse duration.
REFERENCES
1. M. Mielke, “Ultrashort-pulse lasers bring cost-efficient precision to micromanufacturing,” Laser Focus World, 51, 4, 49–53 (Apr. 2015).
2. See bit.ly/SpindelEye.
Tony Karam | Product Line Manager of Laser Optics, Edmund Optics
Tony Karam is Product Line Manager of Laser Optics at Edmund Optics (Barrington, NJ).