Integrated optical modules for quantum communication
The growing demand for data transmission capacity has led to striking progress in integrated optics within the last two decades. While it is impressive to see a hair-thin glass fiber transmitting complete libraries worth of information in a second, it is equally impressive to see the integrated optics that collect and distribute such huge amounts of data before and after transmission.
Now, we face quantum communication as a revolutionary new approach to data transmission. In particular, secure quantum communication (QC) may benefit from the know-how that has been collected over decades in the development of integrated optics. Adapting conventional technology to the requirements of quantum communication makes use of established production technologies and thus allows for mass production at reasonable costs.
What are the requirements of QC? First, we have to accept that QC is about single photons. The information is stored in their quantum states. Generating them is rather easy—pairs of single photons, for example, are generated in nonlinear down-conversion processes where polarization or other properties carry the actual information. Missing a single photon due to losses means losing information. Amplification is not possible, as an individual photon cannot be made more powerful. Copying the photon would mean reading its information, destroying its single quantum state. Detecting single photons is also difficult, as this requires extremely low dark currents. Consequently, the transmission and detection of single photons is quite difficult.
Establishing a German QC network
The transmission of quantum states—for example, in the form of single or entangled photons—offers a form of inherently secure communication. This has attracted the interest of many governmental bodies in various countries. In 2019, the German initiative QuNET started to explore and realize secure QC technologies. QuNET is an initiative of the Fraunhofer-Gesellschaft, the German Aerospace Center, and the Max Planck Society, and is funded by the German Federal Ministry of Education and Research (BMBF). The BMBF is currently funding the QuNET initiative with around 60 million euros. A total funding volume of 125 million euros is planned until 2026 as part of the German federal government’s cybersecurity research agenda. The funding targets technology development along the value chain in Germany to enable the industrial production of relevant components and systems, as well as the establishment of secure infrastructure in Germany and Europe.
The Fraunhofer Heinrich Hertz Institute (Fraunhofer HHI; Berlin, Germany) contributes several technologies to the project: photonic integrated circuits (PICs) for the quantum network, expertise in fiber-optic networks, and quantum key distribution. This includes the entire chain, from key functionalities via integrated optical components to real-time-capable systems for fiber-based quantum communication.
In the first phase, the focus of component development is on quantum cryptography transmitters and on single-photon detectors for future miniaturized receiver chips. On the system side, the focus is on real-time connectivity of QC setups for the provision of secure keys over existing fiber-optic networks. In addition, Fraunhofer HHI provides the classical communication channels via terahertz links, mobile radio, and fiber optics, which are encrypted using QC and thus enable confidential communication.
A test system for quantum communication
A complete quantum communication system (see Fig. 1) has been set up for development and testing of all QC components. It has been built from of-the-shelf components at the Fraunhofer HHI as a test bed for software, protocols, and new components.
One goal within the QuNET project is to develop integrated components for QC based on established technology. The photon source is a strongly attenuated laser on a hybrid PIC (see Fig. 2). The two small white cubes on the left h the two lasers, being followed by integrated optics such as filter and waveguides for polarization coding on the hybrid PIC module.It is based on the PolyBoard technology, using an on-chip micro-optical bench where ultrathin-film elements, nonlinear crystals, polymer interposers, and single-photon detectors can be combined on a photonic integrated chip. For QuNET, the systems employ the polarization coded BB84 protocol and other solutions such as time-bin coding using delayed photons.
The receiver part looks quite similar, but with one important difference: A typical source generates 1017 to 1018 photons per second, which are attenuated to single photons at a reasonable rate. Losses are actually created to achieve single-photon operation. On the receiver side, single photons simply arrive. Therefore, the loss budget is much lower than on the transmitter side.
The actual receiver is a hybrid PIC receiving its signal through an optical fiber. The photons are sent through polarization filters on the PIC and, finally, counted in sensitive detectors.
Photodetector technologies
The detection of single photons is still difficult. The key to standard single-photon detector approaches is cooling to reduce noise. So far, most quantum detectors must be cooled down to below -200°C. The team at Fraunhofer HHI has set the goal of quasi-room-temperature operation from the very beginning. This is not only a condition for regular field operation—it also leads to much higher lifetimes of the components.
Within the QuNET project, two types of single-photon avalanche diodes (SPADs) are developed. One is the classical approach with top-illuminated SPADs. First preliminary results of the developed vertically illuminated SPAD are demonstrated in Figure 3 for room-temperature operation, showing a clear improvement from the initial design with further potential of improvement to be exploited in the next design iterations. The second SPAD type being developed in QuNET is a waveguide integrated photodiode. This approach has the potential to reduce noise due to smaller photodiode volumes. Furthermore, the waveguide-integrated SPADs allow monolithic integration with passive waveguide components for implementing further signal-processing functionalities.The photodiode chips are based on mature indium phosphide (InP) technology and are fabricated in the wafer process line at Fraunhofer HHI, having Telcordia and space-qualified processes. InP components have been designed, produced, and characterized at this institute for more than 35 years.
Integration
The technology of hybrid integration of different photonic functionalities on a single chiplet has been developed in the PolyBoard project. As mentioned before, it uses an on-chip micro-optical bench where active and passive components can be combined on a photonic-integrated chip.
In the QuNET project, this technology will be used to design, manufacture, and characterize a number of different new modules. This includes hybrid integrated receivers for continuous value (CV) and discrete value (DV) quantum key distribution, as well as low-loss passive routing for the distribution of private keys across more than two participants. This hybrid integration approach allows for a combination of optimal material systems for the required functionality, especially with low-loss passive, on-chip waveguiding and efficient photon generation and detection.In other projects, such as the EU-funded UNIQORN, there have been developments of QC modules including a source of photon pairs (see Fig. 4), a source of entangled photons, and quantum-reconfigurable optical add-drop multiplexers (qROADMs; see Fig. 5). As integration allows small form factors, the goal for QC components is to achieve form factors similar to the telecom and datacom components.
On the module level, the researchers use PICs that are based mainly on InP and PolyBoard technology (see Fig. 6). They have designed and manufactured modules for the generation of entangled photons, for the manipulation of QC signals, and for the reception of QC signals. Prototypes of these modules are currently in the test and characterization stages. By the end of 2021, these integrated optical modules will be introduced to the QC system, step by step on a plug-and-play basis.
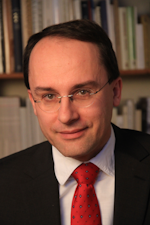
Andreas Thoss | Contributing Editor, Germany
Andreas Thoss is the Managing Director of THOSS Media (Berlin) and has many years of experience in photonics-related research, publishing, marketing, and public relations. He worked with John Wiley & Sons until 2010, when he founded THOSS Media. In 2012, he founded the scientific journal Advanced Optical Technologies. His university research focused on ultrashort and ultra-intense laser pulses, and he holds several patents.
Moritz Kleinert | Project Manager, Fraunhofer HHI
Moritz Kleinert is a Project Manager at the Fraunhofer Heinrich Hertz Institute (Fraunhofer HHI; Berlin, Germany).
Patrick Runge | Head of Detectors Group, Fraunhofer HHI
Patrick Runge is Head of the Detectors Group at the Fraunhofer Heinrich Hertz Institute (Fraunhofer HHI; Berlin, Germany).