Over the last half-century, the power of computers has changed our lives. Industrial entities are still hungry for even more computational power, but classical computers are at their limits. As Jeremy O’Brien, CEO of PsiQuantum (Palo Alto, CA) and a former professor at the University of Bristol (UK), said during a World Economic Forum event for emerging technologies, “Even if we turned our entire planet into a giant supercomputer, we would not be able to solve some of the computational problems across artificial intelligence, in the design of new materials, pharmaceuticals, and clean energy, among others. The good news is that if we could build a computing device based on fundamental quantum principles, we could.”
Quantum computers are unquestionably the future. During the last decade, dozens of companies and startups started spending hundreds of millions of dollars in a race to build the world’s best quantum computer. These devices will provide computational power to solve problems that otherwise would have been impossible (i.e., the quantum advantage). The biggest challenge is scaling the number of available qubits to a level where we can have a practical quantum computer.
Many quantum objects can incorporate the concepts of superposition, entanglement, and interference for performing quantum computing—including those based on superconducting qubits, diamond vacancies, ions, and photons, which are among the most promising. They are currently being explored to harness quantum physics’ power to break down science and industry barriers. Photonic qubits have a dominant place because semiconductor technology can be used to scale up the number of qubits. A hybrid or monolithic approach could potentially be adapted to have large-scale scalable linear optical circuits for routing, processing, and analysis within a single device. Beyond the core technologies, any real quantum device will need enabling technologies such as cryocooling systems, ultralow-noise electronics, and optical switches and interconnections.
Centered on this approach, PsiQuantum was founded in 2016 with the goal of building the world’s first large-scale, fault-tolerant, error-corrected silicon-photonic quantum computer. The fundamental four scaling challenges are manufacturability, control electronics, qubit connectivity, and cooling power. A few years ago, they teamed up with GlobalFoundries (Malta, NY), one of the largest specialist chip production companies, in response to manufacturing challenges and have created a full quantum-photonic fabrication flow to generate, manipulate, and detect quantum states of light within silicon photonic integrated circuits (PICs). The next step is integrating high-detection-efficiency niobium nitride (NbN) thin-film superconducting single-photon detector technology into CMOS fabrication. A $450 million investment is enabling PsiQuantum to leverage the semiconductor supply chain, assembly, manufacturing, and packaging toward building the first practical quantum computer.
Quantum innovations in Europe
On the European side, QuiX Quantum (Enschede, Netherlands), founded in 2019, is working on a specialized photonic quantum computer based on silicon nitride (SiN) PICs, machine learning, and quantum simulation applications (see Fig. 1). They have sold five quantum photonic processors—for a large-scale, fully tunable linear interferometer—to academics and startups, and are collaborating with major universities. Such a device sits at the heart of a photonic quantum computer, and was achieved thanks to the vertically integrated offering at LioniX International (Enschede), a developer of PIC modules. The processor has a record of 12 optical channels, low optical losses (2.5 dB), and high transformation fidelity (98%). PHIX Photonics Assembly (Enschede), a volume packaging foundry, packages the chip and integrates it into a plug-and-play control box, which can be designed to operate at wavelengths between 450 and 2300 nm.
The next step regarding this device is to scale it up in electrical interconnections at wafer-level electrical and optical packaging supported by LioniX and PHIX, respectively. Jelmer Renema, the CTO of QuiX, spoke about a 20-mode quantum processor’s arrival within the following months during the EPIC Online Technology Meeting on Large Scale Qubit Generation on December 1, 2021, and the quantum community is waiting to see if it’s true.
A SiN platform is developing a reputation for performance in quantum applications due to its low propagation losses. The absence of two-photon absorption (TPA) allows high-power operation levels, and photon-pair generation can be achieved via spontaneous four-wave mixing (SFWM). In addition, the resonators’ narrow bandwidth and possible tunability provide a high degree of indistinguishability of generated photons, which is a prerequisite for quantum computing with photons.
LioniX’s TriPleX technology has the lowest propagation losses reported for SiN waveguides (0.1 dB/cm down to 0.1 dB/m). It operates within a broad wavelength band ranging from 405 to 2350 nm, promising high flexibility for customization. LioniX also developed a patented tapering method to convert low-contrast modes for optimal fiber coupling to high-contrast modes for small bending radii. This feature enables very low-loss hybrid integration of the high-performance active-light sources and detectors required for a quantum processor.1
To enable plug-and-play functionality—vital in QuiX Quantum’s processor—LioniX applies a vertically integrated approach to module development. This offering builds on specialized waveguide technology, which is developing alongside driver electronics, interfaces, and housing required for near out-of-the box performance.
Ligentec (Lausanne, Switzerland) offers a foundry service based on SiN technology that enables customized PICs and allows companies and research institutions to fabricate their designs from prototype to volume quantities with excellent process control. Their process ensures exceptional mode confinement, sharp bending radius, reduced device footprint, and ultralow losses (below 0.1 dB/cm).
Achieving high-performance optical technologies is very important for quantum photonic processors. This includes high-performance optical switches, high-performance chip-to-fiber interconnects, and low-loss optical components. Notably, operating larger, more complicated systems at cryogenic temperatures results in a high photonic, electronic, and thermal control level. Enabling technologies include innovative hybrid packaging, co-packaging, and bonding techniques to integrate photonics and electronics together.
Packaging facilities supporting such hybrid approaches are now commercially available (e.g., PHIX, LioniX). In addition, Teem Photonics’ (Meylan, France) WAFT series (waveguide array to fiber transposers) enables high-performance integrated photonic packaging and has widespread use in telecommunication and classical photonic computing. This approach is an excellent solution for coupling quantum light in and out from the quantum PICs.
PIXAPP, which is the world’s first open-access PIC assembly and packaging pilot line, provides a range of standardized photonic packaging technologies that could be scaled to high volumes and training in optical, electrical, and mechanical packaging technologies. PIXAPP offers a menu of packaging processes in the form of building blocks, allowing standardized manufacturing processes to be used across the entire supply chain to produce PICs in the major photonic platforms (InP, SOI, SIN), with standard designs for electro-optical I/O ports and packaging assembly steps.
Generally, photonic quantum processors feed themselves with quantum light. This makes single-photon sources essential. Quandela (Paris, France), Sparrow Quantum (Copenhagen, Denmark), and AegiQ (Sheffield, UK) position Europe ahead globally, with capability to fulfill future requirements of delivering photons with high purity, high indistinguishability, and brightness for interfacing with such photonic quantum computing devices. Their technologies are based on semiconductor quantum dots (artificial atoms), which work as efficient quantum light sources.
AegiQ is developing a full stack of single-photon technologies, including photon sources operating at a wide range of wavelengths ranging from near-infrared (near-IR) to standard telecom C-Band. Their approach will enable large-scale networking and computing and can support a variety of architectures such as photonic quantum processors based on multimode interferometers or multidimensional cluster-state generation.
Quandela, active since 2017, develops full-stack optical quantum computing solutions based on their highly efficient quantum light emitters and optoelectronics modules for optimized device control and single-photon manipulation. An example of these rack-size modules is the active demultiplexer (available for 6 and 12 photons), which is necessary to interface quantum light sources to photonic quantum processors.
Quandela has already sold single-photon source devices and optoelectronics modules to several clients worldwide (EU, Australia, and Russia) and early this year has released Prometheus—the first standalone single-photon source (see Fig. 2). The company is now on the path to building optical quantum computing platforms based on their semiconductor quantum light emitters, with software stack and modular architectures for efficient scale up; they recently teamed up with QuiX Quantum to deliver a reconfigurable quantum computing platform, anticipating France’s national quantum technologies program that its president, Emmanuel Macron, announced. Another enabling technology comes from iPronics (Valencia, Spain). Their quantum field-programmable photonic gate arrays (FPPGA) can incorporate quantum sources and detectors as high-performance blocks surrounding a programmable silicon photonics waveguide mesh, implementing linear transformations.ORCA Computing (London, UK) aims to integrate quantum memory technology into photonic quantum computing systems, giving a slightly different flavor to scaling up processing qubits. ORCA’s philosophy is about part integration and modular components separated with optical fiber. Such an approach will permit trying and leveraging things from the existing telecommunications supply chain.
The company aims to face one of the key problems of single-photon generation: vacuum states. These are simply holes within a stable array of single photons, making the generation of very large lattices of entangled photons extremely challenging. Their approach is to introduce a quantum memory with the ability to store and release single photons on demand and without extra noise. Then, a much more stable and deterministic resource can be delivered by temporal multiplexing.
Such a deterministic quantum light source is a fundamental challenge of scaling up a photonic quantum computing system. Manipulating single photons in real time and using time as a resource for making quantum computing is not easy. For the synchronization of those resources, high-speed electronics as well as very low-loss, high-speed modulators and fast optical switches are important.
The lithium niobate (LiNbO3)-on-insulator (LNOI) platform, with high intrinsic electro-optical and nonlinear properties, offers required functionalities and is the right platform for delivering cutting-edge, ultra-highspeed electro-optics and nonlinear components for applications in quantum technology, telecom, LiDAR sensing, and metrology for a wide range of wavelengths from 350 nm to 5.5 µm. CSEM (Neuchâtel, Switzerland) is set to establish the first open-access foundry on LNOI and provide full system development capabilities to help solve those challenges. LNOI is another promising platform for quantum integrated photonics because entangled photon pair generation is possible.
Quantum innovations elsewhere
A full-stack approach, building quantum systems all the way up through cloud access, is being taken by IonQ (College Park, MD), founded in 2015. The qubits here are atomic ions offering natural indistinguishability and qubit lifetimes within seconds. In addition, an electromagnetic trap suspends the ions above the device surface, decoupling the ions from the thermal environment, making operation at ambient temperature feasible.
Efficient quantum computing needs noninteracting qubits for long memory times and highly interacting qubits for fast gates; ions can bridge this dichotomy. Long-lived atomic states can be transferred into highly interacting motional states and vice versa using lasers, achieving full connectivity across all of the ions within the chain. A gate between any pair of ions within that chain can be generated by switching which ions are being hit by those lasers. By taking advantage of integrated photonics, the optical quantum control system for trapped-ion quantum computers has excellent potential to be more compact, stable, and scalable. Like classical linking of servers within a datacenter, chains of ions in separate quantum processing units (QPUs) can be entangled via photonic interconnects to create distributed quantum systems (see Fig. 3). This creates full modular connectivity and merges all QPUs into a single, robust system that can support the many qubits required for universal quantum computation in the future.Nitrogen-vacancy (NV) quantum computers are like trapped-ion quantum computers, but instead of being trapped in an electromagnetic field, the qubits are embedded in a material gap, such as nitrogen embedded in diamond or silicon carbide. Quantum Brilliance (Acton, Australia), a startup founded in 2019, aims to build room-temperature diamond quantum computers by also running the full stack approach. They aim to deliver single QPUs that can be implemented in existing classical computers for specific tasks. The heart of such a quantum accelerator is NV centers on diamond. A larger number of qubits can be achieved by having an array of these NV centers positioned deterministically into a diamond crystal. The final product is a box with all of the interfaces to be used as one of the elements in a computer.
Many other companies of different sizes and maturity levels are leveraging technology to achieve the quantum advantage. Photonic qubits and integrated photonics are among the most promising technologies the quantum industry hopes to leverage to scale up quantum devices from the current tens of qubits to thousands of qubits. Thanks to swift development of the telecom and datacom industries during the past 10 years, integrated photonics as a technology to make photonic components compact and scalable is becoming mature in terms of design tools and fabrication capabilities, and is now widely used for many applications. A single integrated photonic chip can now contain thousands of discrete photonic devices fabricated by commercial foundries. In addition, the possibility of hybrid PICs and compatible technologies that will permit the generation, manipulation, and detection of single photons in one single device is of high interest.
ACKNOWLEDGMENTS
EPIC wishes to acknowledge its members and collaborators who are always eager to share their ambitions and vision, in particular Arne Leinse and Tom Horner, LioniX; Jörn Epping, QuiX Quantum; Adrien Billat, Teem Photonics; Niccolo Somaschi, Quandela; José Capmany, iPronics; Maksym Sich, AegiQ; Joost van Kerkhof, PHIX; Michael Geiselmann, Ligentec; and Amir Ghadimi from CSEM and PIXAPP. Developing multiple standardized packaging and packaging building blocks, the PIXAPP pilot line aims to help leading companies and SMEs to exploit PIC technologies for improving their current products and/or to transfer their PIC-related research into PIC-based products. PIXAPP has received funding from the European Union’s Horizon 2020 research and innovation program under grant agreement No 731954.
REFERENCE
1. J. M. Arrazola et al., arXiv (Mar. 3, 2021); https://arxiv.org/pdf/2103.02109.pdf.
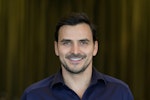
Panagiotis Vergyris
Dr. Panagiotis Vergyris is executive director at Specto Photonics (Milan, Italy). He has a Ph.D. in Quantum Photonics and more than 10 years’ experience in the photonics industry, with technical, managerial, and business roles in different deep-tech companies. Before joining Specto Photonics, he was a photonics program manager at the European Photonics Industry Consortium (EPIC), supporting startups in photonics, orchestrating successful business matches, and supporting and ensuring the viability of the European photonics ecosystem.