‘Impossible’ unipolar terahertz emitter is another step toward quantum computing
A team of researchers led by the University of Michigan and the University of Regensburg built an “impossible” unipolar terahertz emitter to maneuver around the inherent symmetry of light waves. It may enable more asymmetric terahertz pulses, which can be tailored to control semiconductor qubits.
“Applications from computers to cell phones rely on electronics that can switch incredibly fast—about one billion times per second,” says Mackillo Kira, a professor of electrical engineering and computer science at the University of Michigan. “But electronic quantum information in solids is lost typically 10,000 times faster, which so far has prevented everyday electronics from accessing the true power of quantum information processing.”
This inherent slowness of electronic switching can be overcome by using extremely fast electromagnetic waves to switch, characterize, and control electronic quantum states.
Light waves oscillate roughly one million times faster than electronics and may propel next-gen systems to reach petahertz rates—with up to a million billion operations per second. Within this context, an ideal quantum-electronic switch pulse would contain only a positive (or negative) half-cycle of oscillation to flip a quantum state (a qubit) with minimal time (a half-cycle) and maximal efficiency (no back-and-forth oscillations).
Terahertz light falls between infrared (IR) and microwave radiation and, while it oscillates fast enough to provide the necessary speed, the shape of its waves—until now—was an “impossible” problem: purely positive or negative waves aren’t physically possible. All waves consist of positive and negative oscillations that sum to zero.
“We’ve long been inspired to find ways around this wave-shape limitation, to create the shortest possible wave forms to eventually drive petahertz light wave electronics,” says Rupert Huber, a professor of physics at the University of Regensburg. “This aspiration led us to the unipolar wave discovery where a very sharp high-amplitude positive light wave peak is sandwiched between two long low-amplitude negative peaks. The positive peak is strong enough to switch or move electronic states while the negative peaks have almost no affect.”
Building a terahertz emitter
The researchers started with a newly developed stack of nanofilms—each only a few atoms thick—made of different semiconductor materials, such as indium gallium arsenide (InGaAs), epitaxially grown on gallium arsenide antimonide (GaAsSb).
They designed terahertz emission through the motion of electrons and holes, which are essentially the spaces left behind when electrons move in semiconductors.
At the interface between nanofilms, ultrashort IR laser pulses can excite electrons and holes to pull them back together to produce a single-charge oscillation (see Fig. 1).
“The fast-charging and slower-charging oscillations combined to emit a unipolar wave (see Fig. 2), which we tailored as effective half-cycle light pulses in the far-IR spectral region,” says Kira. “In essence, we exploit and control two competing effects—charging and a single-charge oscillation—to control the shape of the pulse electrons and holes emit. First, the ultrafast pulse creates an ultrafast charge separation, followed by the slower and single-charge oscillation. Because these are separate physical processes, we can adjust them to produce unipolar pulses of different durations.”Significant advance
Seamless integration of light waves and electronics are poised to create incredible new possibilities and speeds for digital and quantum information technologies alike.
“Our demonstrated unipolar wave source has the real potential of becoming one of the building blocks for next-gen electronics and light wave electronics,” says Huber. “They could also serve as well-defined clockworks for next-gen ultrafast electronics.”
Beyond enabling the next stages in electronics, the researchers are seeing high demand for ultrashort light pulses in the far-IR and terahertz spectral domain for many other unexpected applications as well.
“Effectively unipolar terahertz fields are a powerful tool to control novel quantum materials on the intrinsic timescales of their microscopic electronic motion,” says Huber. “Moreover, the new emitters are perfectly adapted to operate in combination with modern, industry-grade high-power solid-state lasers and could form an extremely scalable for applications in both fundamental science and industry.”
Advantages of unipolar terahertz emission
The resulting terahertz emission is surprisingly unipolar, and the solely positive half-cycle peaks about four times higher than the two negative field excursions (see Fig. 3).“Our community has long been working on effective ways to produce light pulses with fewer oscillation cycles, but the possibility of generating terahertz pulses so short to effectively comprise less than a single half-oscillation cycle was beyond our boldest dreams,” says Huber. “Because many emitters of the new design can be stacked on top of each other and the lateral area of the emitters is widely scalable, the new source can also be designed for large-peak terahertz field strengths.”
Light waves are poised to revolutionize next-gen electronics via a million-fold boost to their speeds as well as by opening the door to room-temperature quantum applications. “It’s really cool that this future may be quite near,” says Kira.
A few years ago, the researchers flipped quantum information with light waves and now they’re providing a unipolar pulse to do it with extreme precision. The next hurdles will be to clock sequences of quantum information processes to perfectly control them, and eventually integrate these quantum innovations onto a chip.
“We’ve started using these pulses to explore new platforms for quantum information processing,” says Kira.
Furthermore, coupling these pulses into a scanning tunneling microscope “allows us to speed up atomic-resolution microscopy to few-femtosecond timescales and capture real-space and real-time motion of electrons in actual ultraslow-motion microscopic videos,” Huber adds.
RELATED READING
C. Meineke et al., Light Sci. Appl., 11, 151 (2022); https://doi.org/10.1038/s41377-022-00824-6.
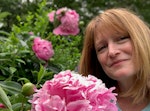
Sally Cole Johnson | Editor in Chief
Sally Cole Johnson, Laser Focus World’s editor in chief, is a science and technology journalist who specializes in physics and semiconductors. She wrote for the American Institute of Physics for more than 15 years, complexity for the Santa Fe Institute, and theoretical physics and neuroscience for the Kavli Foundation.