In a significant quantum advance, University of California – Santa Barbara (UCSB) researchers recently created a method to produce a fast and steady stream of single photons—essential for enabling photonics-based quantum technologies.
Collecting photons efficiently is difficult because quantum emitters can emit single photons in nearly any direction, so they need to be placed into an intermediary medium (think funnel) to be collected and directed onto a specific path of travel.
To do this, the researchers are using an on-chip optical cavity (ring resonator) as their intermediary medium, which collects most of the light from the single photon sources and funnels it to an on-chip waveguide very efficiently.
Putting these single photons into many different waveguides—a thousand single photons on each waveguide—means they can choreograph how the photons travel along the waveguides on chip, which, in turn, enables quantum computation.
Shrinking optical systems onto photonic chips
The quantum photonics field has been developing along with other competing platforms for years, “but maybe with less hype,” says Kamyar Parto, a Ph.D. student working with Galan Moody, a quantum photonics expert and assistant professor of electrical and computer engineering at UCSB.
“In the past few years, researchers have demonstrated bulk tabletop optical systems capable of achieving a quantum advantage for specific tasks, demonstrating the capabilities of photonics for quantum computing,” says Moody. “But ultimately, these optical systems need to be shrunk down onto photonic chips that can be networked together to become scalable and manufacturable.”
Quantum computing platforms rely on defining a qubit, doing operations on qubits, and then measuring their quantum states. For a quantum photonics approach, quantum information is encoded onto the properties of single photons, such as the orientation of their electric field, frequency, or their path of travel.
“For quantum photonic computing, it’s then a matter of generating streams of high-quality single photons with high efficiency, making different streams of photons interact with each other to carry out computations, and then detecting the photons,” says Moody.
This can all be achieved via tabletop setups and routing photons around using mirrors and other optical components (see Fig. 1), but Parto points out it’s possible to run out of room on your optical table quickly. “Integrated quantum photonics allows you to bring almost everything you can do on an optical table to a chip, where everything is condensed and stable,” he says. “For instance, you can route the light to specific locations on the chip using waveguides, where other components then act to manipulate the properties of the photons or detect them.”
Generating high-quality photons via 2D materials
Since single-photon sources in two-dimensional (2D) materials were discovered about eight years ago, the quantum community has made progress characterizing, controlling, and engineering these sources.
While most materials we’re familiar with can grow their crystal in all 3D of space, 2D materials like graphene and tungsten diselenide only have 2D atomic bonds, which makes them extremely thin. It’s the inherent thinness and confinement in one direction, Parto says, that can make these materials unique in their electro-optical properties.
“The defects in these materials are atomic point defects,” he explains. “They can be as simple as just one missing atom from the crystal and, because of this, they go beyond the diffraction limit and can’t be seen with a normal microscope.”
But the researchers can identify their position by scanning a tiny laser spot (<300 nm) over the materials to look for the optical signatures of the defect (see Fig. 2).“2D materials have remarkable properties, such as the ability to operate at room temperature,” says Parto. “These materials can also be integrated with nearly any other material system, which opens up lots of opportunities—not only in quantum information and communications, but also sensing, optoelectronics, biology, and chemistry.”
For these materials to be useful for quantum information, it’s important to collect as many emitted photons as possible. A major hurdle for quantum photonics, however, is generating high-quality photons in the first place, and collecting and guiding these photons on a chip efficiently. “This is the focus of our project using a relatively new material system based on 2D crystals,” says Parto, who is working toward his Ph.D. on engineering defects within 2D semiconductor materials.
There are many ways to generate single photons, but the Moody Lab researchers are crafting defects within certain 2D semiconductor materials that are only one atom thick (see Fig. 3). To create a defect, they essentially remove a tiny amount of material.When you shine laser light onto one of these defects, the material responds “by emitting single photons,” says Parto. “The defect acts as a rate-limiting state, which allows it to behave like a ‘factory’ for pushing out single photons, one at a time.”
One big advantage of 2D materials is they lend themselves to having defects engineered into them. Further, Parto says “these materials are so thin, you can pick them up and put them on any other material without being constrained by the lattice geometry of a 3D crystal material. This makes the 2D material very easy to integrate.”
Alignment
One of the coolest aspects of this work for Parto was aligning the position of the quantum light source, which is an atomic defect within the material, to the center of the 600-nm-wide waveguide with sub-100-nm precision.
“There’s almost no room for error,” he says. “For the alignment, we used a relatively inexpensive and custom-made optical microscope to identify the position of the quantum defects. But at that scale, the optical image distortions usually make any atomic-scale alignment impossible. With a few little tricks, we corrected most of the image distortions and achieved the accuracy we needed. Everyone was surprised we could still do so much with relatively simple and old technology.”
Ultimate goal
The ultimate goal for single-photon sources “is a device where you can push a button and a single photon would shine into your waveguide with perfect efficiency,” says Parto. “All the metrics we care about revolve around this concept. For instance, you want to ensure that every time you push a button you get a single photon into the mode—no misses allowed. You want all photons to have the same phase and frequency, to be indistinguishable from each other.”
The quantum photonics community is advancing fast on all of these fronts and, “the next step for us is to realize another requirement: to make tens to hundreds or thousands of these devices on a chip,” he adds.
At this scale, it becomes impractical to trigger these single photons using lasers, as Moody’s lab is doing now. “To make things scalable, we need to create single-photon light-emitting diodes (LEDs) we can trigger with electrical signals,” says Parto. “This is a special type of LED because light from LEDs generally shine everywhere, but we want a single-photon LED that shines solely into the unique mode of a waveguide. Our previous work is a stepping stone toward this, and now we hope to show that creating such a complicated electro-optical device is possible by leveraging the unique properties of 2D materials.”
FURTHER READING
K. Parto et al., Nano Lett., 22, 23, 9748-9756 (2022); doi:10.1021/acs.nanolett.2c03151.
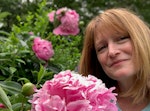
Sally Cole Johnson | Editor in Chief
Sally Cole Johnson, Laser Focus World’s editor in chief, is a science and technology journalist who specializes in physics and semiconductors. She wrote for the American Institute of Physics for more than 15 years, complexity for the Santa Fe Institute, and theoretical physics and neuroscience for the Kavli Foundation.