Entangled photons are so intricately linked that any action on one of the pair affects the other photon—no matter where they are within the universe. It’s a powerful effect of quantum mechanics, and it governs the quantum realm.
A team of researchers from Max Planck Institute for the Science of Light (Erlangen, Germany) and Sandia National Laboratories (Albuquerque, NM) reported a metasurface device to trigger entanglement in complex ways not previously possible in 2022, and they’re continuing their work (see video). Metasurfaces are manmade surfaces designed to interact with light and other electromagnetic waves in ways conventional materials can’t—and they require less space and can do more with light than a traditional optical element.
Quantum state engineering is largely based on the creation of photon pairs, which is traditionally achieved via one of two nonlinear effects: Spontaneous down-conversion or spontaneous four-wave mixing in bulk optical elements. These nonlinear effects cause one- or two-pump photons to spontaneously decay into a photon pair.
The team’s metasurface is an array of notched cube-shaped nanoscale elements—meta-atoms—made of gallium arsenide (GaAs), which is a semiconductor material coated on a 500 µm glass substrate (see Fig. 1). Remarkably, with a laser beam sent through it, the metasurface is designed to produce “complex webs” of entangled photons pairs at a time—even several pairs entangled together—and at different wavelengths (see Fig. 2).
Maria Chekhova, a physicist well known for her quantum optics research who runs the Chekhova Research Group at the Max Planck Institute for the Science of Light, is deeply entangled in this work.
Before this project began, Chekhova focused primarily on quantum optics and generating quantum states of light via nonlinear effects in bulk crystals or fibers. In these types of experiments, the high nonlinearity of the material and its ability to satisfy what’s known as the “phase-matching condition” are critical. But very few materials offer both properties.
Chekhova was drawn to the nanoworld of ultrathin films and metasurfaces by the idea: “What if we sacrifice the phase-matching condition for high nonlinearity? It would allow us to use strongly nonlinear materials never used before,” she says. “The key is to take the material so thin that the phase-matching condition is fulfilled automatically.”
And it turns out, in the future, nonlinear metasurfaces can eliminate the need for the incredibly complex optical setups—a room full of equipment—traditionally used.
Generate quantum states
The quantum states the team generates are mostly pairs of entangled photons. Like other spontaneous effects, such as spontaneous emission of photons by atoms, emission of photon pairs is caused by quantum vacuum fluctuations.
“A metasurface has resonances with a spectral position defined by the shape of its meta-atoms,” says Chekhova. “These resonances enhance the vacuum field and, therefore, the photon emission at the resonant frequencies.”
One of the key things to know about metasurfaces is they’re multifunctional because they can simultaneously support several nonlinear effects, or linear and nonlinear effects at the same time.
“So far, we’ve demonstrated the presence of several channels of photon-pair generation at once, which can be used to form complex quantum states,” says Chekhova. “And another great thing is the enhancement of the pair generation rate compared to an unpatterned film. We achieved greater than three orders of magnitude in this work, and two orders of magnitude in our earlier work.”
Challenges to overcome
The main challenge for the team to overcome is the low rate of emission. For metasurfaces, the amount of material involved in the nonlinear process is miniscule—not more than 100 cubic micrometers—and this makes it difficult to compete with bulk sources.
“Resonances help, but can’t compensate for the tiny size,” Chekhova points out. “So far, we’ve achieved measurable rates of pair emission—a few counts per second—by optimizing the collection of photons, and, of course, by using strongly nonlinear materials and high-quality resonances.”
But these rates are still far too low, so the team, in collaboration with the group of Igal Brener from Sandia, will continue its works to increase them by several orders of magnitude by properly engineering the metasurfaces and choosing even better materials or the same materials, like GaAs, with different orientations.
Another challenge is photoluminescence, which becomes significant as the source of photon pairs gets smaller and contaminates the quantum state. “We recently proposed a remedy: Using a pulsed regime of generation and time-gated detection,” Chekhova says. “Photoluminescence is a slow process while generation of pairs is almost instantaneous. The former can be eliminated by looking at very short time intervals during the pump pulse.”1
The spookiest and not fully understood aspect involved within this realm is the directivity of emission. “Sometimes the photon pairs are emitted forward along the pumping light, sometimes backward, sometimes bidirectionally—one photon forward, the other backward,” Chekhova explains. “Such behavior is different from what we observe in nonlinear optical effects or solid-state emitters in metasurfaces.”
One truly great a-ha! moment occurred when Chekhova and colleagues realized they were looking in the wrong direction for photons emitted forward and discovered pairs emitted backward.
Target: Increase efficiency
Standard quantum applications like quantum key distribution, sensing, and imaging are all still in need of higher emission rates. “The future of metasurfaces as sources of quantum states stems from their multifunctionality,” says Chekhova. “On the same metasurface platforms, for instance, we can generate pairs, impart some quantum features to them like orbital angular momentum, implement frequency conversion, maybe detection, and even quantum gates.”
The goal now is to increase efficiency. “We also plan to generate other quantum states, not only pairs but also squeezed light and photon triplets,” Chekhova says. “And we’ll work to implement several effects at once.”
Quantum photonics is expected to enable new forms of encryption for highly secure channels of communication and new types of supercomputers.
REFERENCE
1. V. Sultanov and M. Chekhova, arXiv (physics.optics); https://arxiv.org/abs/2307.04855.
FURTHER READING
T. Santiago-Cruz et al., Science, 377, 6609, 991–995 (Aug. 25, 2022); https://doi.org/10.1126/science.abq8684.
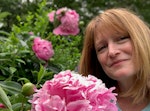
Sally Cole Johnson | Editor in Chief
Sally Cole Johnson, Laser Focus World’s editor in chief, is a science and technology journalist who specializes in physics and semiconductors. She wrote for the American Institute of Physics for more than 15 years, complexity for the Santa Fe Institute, and theoretical physics and neuroscience for the Kavli Foundation.