Physicists discover surface signature of higher-order topological insulators
Physicists from the University of Illinois Urbana-Champaign and Université Paris-Saclay recently theoretically uncovered an unmistakable surface signature of an elusive class of materials—higher-order topological insulators (HOTIs; see video).
Topological insulators are known for their ability to carry electrical currents on their surfaces while possessing insulating interiors. But in HOTIs, electrical conduction is restricted to one-dimensional (1D) edges or “hinges” rather than the entire two-dimensional (2D) surface (see figure). Hinge state conduction can exist both within ordinary materials as well as HOTIs with an unusually high degree of symmetry—which implies their crystal structure must be unrealistically perfect.
“We were trying to find smoking-gun observable signatures of HOTI materials that are robust to imperfections and disorder at the surface,” says Barry Bradlyn, a physics professor at the University of Illinois Urbana-Champaign. “We wanted to look for electronic and optical properties of HOTIs that can be used to identify them, and that could also be used one day in future applications.”
Charles Kane, one of the discoverers of topological insulators, introduced an apt analogy for standard topological insulators, which can help to explain the differences between them and HOTIs. “He said we can think of standard topological insulators as Hershey’s kisses—a conducting metal foil wrapped around an insulator that doesn’t conduct electricity,” says Benjamin Wieder, a junior faculty member at the Institut de Physique Théorique, Université Paris-Saclay. “The chocolate, in this case, is still a pretty good way to understand them. But with HOTIs, it’s as though someone took the foil and crumpled it into a thin ring encircling the chocolate.”
HOTI surface signature detection
To detect a HOTI surface signature, the physicists set out to explore how electrons of different spin behave within a HOTI.
Before their work, most studies on HOTIs focused “either on properties that don’t connect easily with experimental observables, or on extrinsic surface and hinge states that are sensitive to disorder and symmetry breaking,” Bradlyn says.
Instead, the team turned their attention from the hinge state to the interior, where electrons tend to delocalize from individual atoms and spread throughout the entire material. Most importantly, the researchers considered differences in spin—which allows electrons to behave as miniature magnets.
When they divided the interior electrons into their two possible states, up and down, “we saw that each state leaves a unique surface signature,” says Kuan-Sen Lin, a physics graduate student working with Bradlyn. “Although the surface of a HOTI seems uninteresting, when you look at what each spin is separately doing on the surface, an unmistakable behavior emerges that we hope will soon be measured in an experiment.”
Electrons with different spins behave as magnets, so they respond differently when electric voltage is applied to the material, causing the two spin states to accumulate on opposite sides. The magneto-optic Kerr effect, in which the polarization or orientation of light changes when it reflects from the surface of a magnet, can be used to detect this accumulation.
The physicists showed mathematically that electrons in a single spin state within a HOTI behaved as if they were moving inside a magnetic material with a known electromagnetic response. “Using this intuition, we mathematically calculated a ‘spin-resolved’ response at the surface of the material that’s half of what you would expect for an ordinary 2D surface,” explains Bradlyn. “This is exciting because it gives the first prediction for a robust experimental signature of HOTI materials.”
They hope their work shows that the insides and surfaces of topological materials “still host many mysterious and advantageous features—if you know how to look for them,” Bradlyn adds.
And, in fact, they know where to start looking: the team identified bismuth bromide, a well-known insulator, as a strong candidate to observe this effect.
Old ideas put the HOTI puzzle together
Most thrilling aspect of this work for the team? “It was when we first realized the idea of looking at spin-resolved properties can be applied to study HOTIs,” says Bradlyn. “Spin-resolved band topology was originally introduced about 15 years ago by Emil Prodan to resolve questions about spintronic response in 2D topological insulators, but then was quickly pushed aside and forgotten.”
When Lin came across Prodan’s work and implemented it numerically to benchmark some finite-size calculations on a HOTI model, they realized “these old ideas had something very interesting to say about HOTIs,” Bradlyn says. “It felt like several pieces of the puzzle immediately came together, and after that we just had to work out the details.”
The main challenge the team faced was putting together theoretical formalism, numerical calculations, ab initio simulations of material properties, and experimental proposals.
If you’re wondering if quantum applications are ahead, “the properties of HOTIs we identified here could be very useful in quantum computing and spintronic devices, but we need to see them in experiments first,” Bradlyn says.
The team is “currently trying to extend our formalism to analyze topological crystalline insulators protected by other symmetries, as well as looking at superconducting systems,” Bradlyn adds.
“There are thousands of topological crystalline insulators like bismuth bromide that were thought to have trivial electromagnetic response,” says Wieder. “This study represents just the tip of the theoretical iceberg in terms of identifying advantageous responses in real-material HOTIs and topological crystalline insulators, and we’re tremendously excited for what comes next.”
FURTHER READING
K.-S. Lin et al., Nat. Commun., 15, 550 (2024); https://doi.org/10.1038/s41467-024-44762-w.
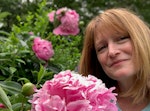
Sally Cole Johnson | Editor in Chief
Sally Cole Johnson, Laser Focus World’s editor in chief, is a science and technology journalist who specializes in physics and semiconductors. She wrote for the American Institute of Physics for more than 15 years, complexity for the Santa Fe Institute, and theoretical physics and neuroscience for the Kavli Foundation.