Technology Review: Laser Focus World's top 20 photonics technology picks for 2016
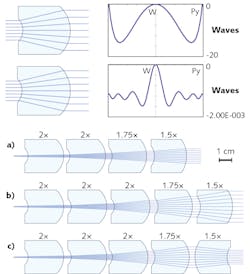
This year, two decades of laser, optical, vibration isolation, and instrumentation development on the Laser Interferometer Gravitational-Wave Observatory (LIGO) bore fruit, resulting in the launch of a new form of experimental science (gravitational astronomy). As a result, this year's Tech Review Top 20 list concludes with an especially robust selection of science topics from the past year of Laser Focus World. And, as always, we are featuring our choice technology picks from this past year's advances in photonics.
Ingenious optics
1. Antireflection nanotextures for laser optics go commercial. With their potential for very wide bandwidths and simplified manufacturing processes, nanotextured thin-film antireflection (AR) surfaces, which come in both randomly patterned and periodic-array forms, have been the subject of much R&D over the years—notably at TelAztec (Burlington, MA). Now, TelAztec and Edmund Optics (Barrington, NJ) have partnered to commercially introduce randomly patterned nanotextured AR coatings on Edmund's laser optics. Because no dissimilar materials are used in the fabrication of these coatings, they have no absorption, reducing thermal-lensing problems and raising the laser-damage threshold. (See "Laser Optics: Antireflection nanotextures for laser optics go commercial," September 2016 issue; http://bit.ly/2dP2qOt.)
2. Monolithic afocal beam expanders. Conventional laser beam expanders have not changed much in design over the years, as they do what they need to do, and do it well. However, new approaches are always welcome, especially when they bring something new to the table. In the case of a type of beam expander introduced to the market by Asphericon (Jena, Germany), the new characteristic is that the beam expander is actually a set of individual monolithic aspheric beam-expanding elements (meaning that each element is a thick lens that serves as a Galilean telescope and thus a complete beam expander) that can be put together in cascades, and can even be reversed, to create many different beam-expansion ratios (see Fig. 1). A separate adjustable triplet can be added to correct for wavefront aberrations induced by changes in wavelength. (See "Laser Optics: Monolithic afocal beam expanders provide flexible capability," January 2016 issue; http://bit.ly/1SB9XkC.)
3. Practical metamaterial optics. There were several examples this past year of metamaterial lenses and mirrors that are well enough developed that they could be used for real-life applications. A high-efficiency achromatic reflective metasurface developed at Shenzhen University (Shenzhen, China) and the University of Birmingham (Birmingham, England) focuses light at three wavelengths across the visible spectrum over an incidence-angle range of 10° to 80°; a planar visible-reflection metalens developed at Harvard University (Cambridge, MA) and the University of Waterloo (Waterloo, ON, Canada) has a numerical aperture (NA) of 0.8, operates at three wavelengths, and is meant for use in microscopy; and a low (0.05) NA monochromatic metalens also produced at Harvard and the University of Waterloo that produces two high-quality monochromatic images separates light into its left- and right-circularly polarized components. (See "These metalenses are actually practical," Laser Focus World online [June 24, 2016]; http://bit.ly/2fosxvY.)
Pushing limits with light
4. 400 W green-emitting thin disk laser. Via intracavity second-harmonic generation, a 130-μm-thick, 15-mm-diameter, 10 atomic percent (at. %) ytterbium-doped YAG (Yb:YAG) disk laser provided by Trumpf (Ditzingen, Germany) was modified by scientists at the Institut für Strahlwerkzeuge, Universität Stuttgart (Stuttgart, Germany) to produce 403 W of light at a 515 nm wavelength with a beam quality (m2) of <1.35 up to 300 W of power and 1.9 above 300 W. The disk was mounted on a diamond heat sink and diode-pumped at a 969 nm wavelength; a 15 × 4 × 4 mm lithium triborate (LBO) crystal placed in the cavity frequency-doubled the light at a 40.7% efficiency. (See "Green thin-disk laser emits 400 W CW in a near-fundamental mode," January 2016 issue; http://bit.ly/1QH5re0).
5. Frequency-doubled and quadrupled laser diodes produce deep-UV light. While laser diodes are normally limited at their short-wavelength end to wavelengths only slightly shorter than the visible range, a group at Toptica (Munich, Germany) has twice frequency-doubled the light from external-cavity diode lasers (ECDLs) to reach wavelengths below 193 nm by using potassium fluoroboratoberyllate (KBBF) crystals mounted between prisms to allow the crystals to reach their phase-matching angle without total internal reflection. The 193 nm version has a peak power of 15 mW with a linewidth below 1 MHz and a mode-hop-free frequency tuning range of more than 80 GHz for VUV spectroscopy. Other uses of such lasers include inspection for deep-UV semiconductor lithography. (See "Novel Lasers: Short, shorter, shortest-Diode lasers in the deep ultraviolet," June 2016 issue; http://bit.ly/2eO7fd7.)
6. Visible light pulses in the attosecond range. Conventionally, the shortest laser pulses in the visible spectral region used by scientists have been in the 2–3 fs range. Now, researchers at the Max Planck Institute for Quantum Optics and Ludwig-Maximilians University (both in Garching, Germany), Texas A&M University (College Station, TX), and M. V. Lomonosov Moscow State University (Moscow, Russia) have used a so-called light-field synthesizer to create pulses in the visible-light region only 380 attoseconds long. The group is headed by Eleftherios Goulielmakis, who pioneered high-harmonic-generation attosecond-pulse technology and science. However, the light-field synthesis approach used here is different from Goulielmakis' other methods. (See "Attosecond Technology: Visible-light pulses are only 380 attoseconds long," March 2016 issue; http://bit.ly/2fB2AKe.)
7. Going way shorter—to zeptoseconds. Researchers at the University of California at Irvine and DER-IZEST, École Polytechnique (Palaiseau, France), in collaboration with scientists at the Extreme Light Infrastructure Nuclear Physics (ELI-NP) facility in Magurele, Romania, are planning to use a petawatt laser in combination with a relativistic plasma mirror to compress near-infrared (near-IR) single-cycle laser pulses to the zeptosecond (zs) regime (see Fig. 2). To produce pulses in the zs range, top-hat-profile 15 J single-cycle near-IR pulses will meet a laser-driven plasma mirror created and propelled toward the pulse at relativistic speeds. Applications will include efficient laser ion accelerators, on-chip tera-electron-volt particle accelerators, and even light materialization in a vacuum, where the zeptosecond pulse actually breaks the bonds between "virtual" elementary particles to create real ones. (See "High-energy Lasers: Extreme light in zeptoseconds," April 2016 issue; http://bit.ly/2fFUMHw.)Imaging with weak inputs
8. CMOS camera with 1000X higher dynamic range. The complementary metal-oxide semiconductor (CMOS) imaging camera is under continual improvement, allowing it to handle some imaging tasks that were the exclusive domain of the charge-coupled-device (CCD) camera. Now, researchers at University College Cork (Cork, Ireland) have created a CMOS camera that has a three-orders-of-magnitude (or factor of 1000) improvement in dynamic range when compared to a conventional CMOS camera—they call it a coded-access optical sensor CMOS (CAOS-CMOS) camera. The new camera uses electronic and optical pixel manipulation in both the space and time/frequency domain to form "agile" pixels that act on selected regions of the image to observe unseen high-contrast features, which results in a dynamic range of 82 dB—with upcoming modifications to further improve this figure. (See "CMOS Cameras: CAOS-CMOS camera has 1000X better dynamic range," August 2016 issue; http://bit.ly/2emJYjV.)
9. Always-on gesture-recognition camera. For remote battery and/or solar-powered imaging applications related to Internet of Things (IoT), security, and other uses, cameras that are capable of very low-power operation are important—especially desirable would be cameras (and associated electronics) that "wake up" and operate only when needed. A group at the Georgia Institute of Technology (Georgia Tech; Atlanta, GA) not only achieves these goals, but does this smartly with a camera that tracks motion in a low-level, generalized (non-pixel-by-pixel) way that still preserves crucial details about what was being tracked. For example, to wake the camera, a person could simply write a "Z" in the air. (See "Low-power gesture-recognition camera is always on," Laser Focus World online [August 16, 2016]; http://bit.ly/2gKhlxh.)
New approaches to old problems
10. Algorithmically designed integrated photonics devices. Engineers designing a component for any kind of system, whether it be optical, mechanical, electronic, or a combination of these, have traditionally approached this task in the same way: 1) come up with a concept based on smarts and experience; 2) refine the concept through calculations, discussions, and so on; 3) test; and 4) possibly repeat one or more of these steps. However, modern iterative, genetic, and artificial intelligence (AI) computer algorithms provide an alternate approach: present the parameters and let the algorithm find the solution. This approach now works stunningly well for integrated photonics, as demonstrated by researchers at Stanford University (Palo Alto, CA) and other groups. The results, however, can look a bit bizarre (see Fig. 3). (See "Silicon Photonics: Design approach to integrated photonics explores entire space of fabricable devices," March 2016 issue; http://bit.ly/2fGf0PE.)11. Radiation-pressure measurement of beam power. Measuring the power of high-power laser beams traditionally gets more difficult as the beam power gets higher, for the simple reason that the beam, which is often intended for materials processing, becomes capable at high-enough power of processing the material of the beam-characterization device. But what if there were approaches that actually became easier as the beam power grew? Scientech (Boulder, CO) has unveiled a laser power meter that takes such an approach. Based on the measurement of radiation pressure produced as the beam bounces off a mirror, the instrument has been tested at a 500 kW incident beam power, and could, with the right mirror, go much higher. The method is practical for beam powers of 10 kW and up. (See "Beam Characterization: Laser power meter measures 500 kW using radiation pressure," September 2016 issue; http://bit.ly/2fFYDXf.)
12. Terahertz-rate data over optical fiber. Because of water-vapor absorption, wireless communications in air using terahertz radiation is relatively short-range. But mixing terahertz and optical radiation in nonlinear crystals allows the signal to be carried long-range—for example, over optical fiber. However, the frequency sidebands produced can interfere with one another and clutter the signal. Researchers at Radboud University (Nijmegen, Netherlands) have now come up with an optical single-sideband (OSSB) modulator that eliminates this problem. Uses will include transmitting ultra-high-definition and virtual-reality images. (See "Optical single-sideband modulator allows terahertz-rate data transmission over optical fiber," Laser Focus World online [October 5, 2016]; http://bit.ly/2eO5blc.)
Photonics and science
13. The incredible LIGO laser/optical system.Along with the rest of the world, Laser Focus World announced that the twin Laser Interferometer Gravitational-wave Observatory (LIGO) detectors, located in Livingston, LA, and Hanford, WA, directly detected gravitational waves for the first time (see Fig. 4). The results allowed us humans to accurately characterize a black-hole merger that happened 1.3 billion years ago. But the true story is the extensive R&D that made the discovery possible. The optical system and laser for the LIGO interferometer themselves are the culmination of a remarkable design process that resulted in some very finely honed hardware. For example, the LIGO mirrors are heated by the main laser and thus deform—to counteract this, light from a carbon dioxide (CO2) laser is distributed precisely across the mirrors to cancel out the thermal deformation. (See "CO2 laser precisely counter-heats LIGO main mirror," April 2016 issue; http://bit. ly/2emKzCb.)14. Yes, plasma mirrors exist. For those whose curiosity was piqued by the mention of laser-produced plasma mirrors potentially used for the production of zeptosecond-scale laser pulses, laser-produced plasma mirrors have already been produced (although not traveling at the relativistic speeds needed for zeptosecond pulse creation). Researchers at Heinrich-Heine-Universität Düsseldorf (Germany) have used oppositely propagating laser pulses to generate a transient plasma photonic crystal (TPPC) consisting of arrays of periodic microplasma sheets—in effect, a plasma thin-film mirror or filter. According to the researchers, for femtosecond to picosecond pulse durations, the TPPC structures can handle fluence values more than 100,000 times larger than conventional laser-damage thresholds on the order of 10 J/cm2. (See "Transient plasma mirror/filter manipulates high-energy laser pulses," September 2016 issue; http://bit.ly/2eWyCPM.)
15. Fast photonic neural networks. Will photonics help enable the upcoming vision of a highly automated, Internet- and robot-served society based on AI? Without a doubt it will, as evidenced by a prototype of an all-optical neural network developed at the Massachusetts Institute of Technology (MIT: Cambridge, MA) and Twitter (also in Cambridge) that is potentially 100 times faster than electronic versions. While a neural network must ultimately do both linear and nonlinear operations to be fully capable, the prototype did its linear operations optically (using a network of waveguide-based Mach-Zehnder interferometers) and its nonlinear operations via software. Future versions will use nonlinear optical devices so that all computations will happen via light alone. (See "Integrated Optics: All-optical neural network can be 100 times faster than electronic versions," November 2016 issue; http://bit.ly/2fkV47D.)
16. Optical amplification for telescopes. All of optics has to contend with the diffraction limit. Astronomy is not excluded: for any optical telescope, approaching the diffraction limit—often, for ground-based telescopes, by using adaptive optics—is the ideal. However, according to researchers at the University of Cambridge (Cambridge, England) and the Technion-Israel Institute of Technology (Haifa, Israel), the diffraction limit applies only to independent photons—with sets of coherent or entangled photons, the limit can be smaller. They propose a telescope based on stimulated emission, in which excited atoms are placed within the telescope. Stimulated-photon bursts only above a certain size would need to be used, which would reduce sensitivity, necessitating longer exposure times. The researchers even fly the idea of imaging exoplanet surfaces. (See "Optical amplification for large astronomical telescopes could overcome the diffraction limit," Laser Focus World online [July 6, 2016]; http://bit.ly/2emOCyf.)
Photonics molds the human body
17. Reattaching nerves with lasers.One of the more-challenging tasks in the biomedical arena, and one that's generating huge amounts of research, is the transplant or reattachment of human hands, limbs, faces, and so on so that the recipient regains at least some nerve regeneration, which leads to recovery of the ability to sense via feel. The same challenge exists for less-major injuries in which a nerve is severed. While nerves can naturally regenerate, what's needed is a more deterministic method to reattach nerves. A group at the University of Alberta (Edmonton, AB, Canada) is now using a near-IR femtosecond laser to perform "hemifusion" of healthy soma (the main nerve cell body) and axons (the extensions from the cell body that connect to other soma, dendrites, or axons). The pulses produce cross-linking that forms the needed strong, shared phospholipid bilayer. (See "Femtosecond lasers weld neurons back together," April 2016 issue; http://bit.ly/2fGfOnE.)
18. Investigating heart cells via micromanipulation. The study of heart-muscle cells called cardiomyocytes, including its mechanical functioning, is important to the understanding of cardiovascular disease. Optical microscopes containing mechanical manipulators can be used to test myocytes' auxotonic contractions (myocyte shortening) and isometric forces (which don't involve physical shortening)—the finer the capability to manipulate, the more knowledge is gained. The MyoStretcher system from IonOptix (Westwood, MA) allows highly sensitive force and motion measurements down to the cellular level, allowing single myocytes to be picked up, moved around for best viewing, and electrically stimulated. Two x-y-z micromanipulators from Siskiyou (Grants Pass, OR) provide the motion capabilities. (See "Cell Biology/Cardiology: Ultra-stable micromanipulators enable nanoscale force measurement," September 2016 issue; http://bit.ly/2fFZpRX.)
19. Noninvasive refractive surgery. Laser-assisted in situ keratomileusis (LASIK) refractive eye surgery has allowed millions to discard their eyeglasses (or at least switch from trifocals to bifocals). However, in LASIK, before laser-assisted corneal shaping, the outer eye covering has to be cut and peeled back to allow access—an obviously invasive procedure. An alternative developed by scientists at the University of Rochester and Clerio Vision (both in Rochester, NY) is based on using a femtosecond laser to induce refractive-index change (LIRIC) within the cornea entirely noninvasively. Surprisingly, the procedure also works on contact lenses and the intraocular lenses (IOLs) that surgeons use to replace the clouded lens removed during cataract surgery. After finding the needed correction, a compensating optical profile, which can have an arbitrary shape, is written into the cornea via LIRIC. Human trials (on blind participants at first) are planned. (See "Ophthalmology/Femtosecond Lasers: LIRIC-Next-generation refractive laser surgery," November 2016 issue; http://bit.ly/2gacNMX.)
20. Superresolution microscopy sees to 5 nm. Superresolution microscopy has revolutionized biomedical microscopy, allowing scientists to image biological structures at resolutions far below the diffraction limit. This year, researchers from Harvard University (Cambridge, MA) have pushed the limits of this technology, developing what they call discrete molecular imaging (DMI), which allows DNA features positioned only 5 nm from each other in a densely packed, single molecular structure to be discriminated (see Fig. 5). The technique is based on short DNA strands that repeatedly bind and unbind, creating easily discerned blinking at a rate that is unique to each chosen short strand. DMI complements current structural biology methods like x-ray crystallography and cryo-electron microscopy, say the researchers. (See "Method pushes super-resolution microscopy to distinguish individual features in single molecules," BioOptics World online (July 6, 2016); http://bit.ly/2eG4tTJ.)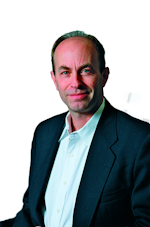
John Wallace | Senior Technical Editor (1998-2022)
John Wallace was with Laser Focus World for nearly 25 years, retiring in late June 2022. He obtained a bachelor's degree in mechanical engineering and physics at Rutgers University and a master's in optical engineering at the University of Rochester. Before becoming an editor, John worked as an engineer at RCA, Exxon, Eastman Kodak, and GCA Corporation.