Ultrafast Laser Optics: Femtosecond laser optics combat pulse dispersion, color errors, and reflections
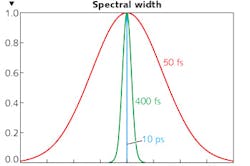
GÜNTER TOESKO and CHRISTIAN DEHNERT
The pace of the development of ultrafast lasers—also called ultrashort pulse (USP) lasers—has been extremely rapid. Picosecond and femtosecond laser systems are efficient tools in many industrial and scientific applications, offering useful nonlinear effects and "cold ablation" for a reduced heat-affected zone (HAZ) that reduces or even eliminates post-processing cleanup in materials processing applications.
Besides multiphoton absorption and self-focusing in transparent materials for improved outcomes in ophthalmic (Lasik surgery) and biological applications, the nonlinear attributes of ultrafast lasers are also used to modify the index of refraction and distribute the laser energy along the beam axis to maintain a near-constant beam radius over many Rayleigh lengths, enabling a very large depth of field.
As a result, USP lasers are well suited to contour cutting (filament cutting) of uncured, chemically hardened glass (cover glass of smartphones) and sapphire, resulting in very high-quality edges and very little material removal. This plasma dissociation process leads to cutting kerfs smaller than 1 μm-much smaller than the diffraction-limited laser spot diameter.
So, what are the optical challenges with ultrafast lasers? Avoiding dispersion, color shifts, and ghost reflections in ultrafast laser optics are of primary importance in maintaining optimum system performance.
Dispersion
Material dispersion in ultrafast laser optics leads to temporal broadening of the laser pulse by introducing a frequency-dependent delay of the different spectral components of the pulse. The higher the refractive index of a material, the higher the dispersion. In addition, the dispersion effect is greater for shorter wavelengths. For example, a 400-fs-long pulse with a central wavelength of 355 nm suffers a temporal broadening of approximately 0.3 fs while traveling through a 20-mm-thick fused-silica window.
In long-pulsewidth laser beams, the wavelength bandwidth is very narrow and typically no compensation is required in the lens. But as the pulse width shortens, the wavelength spread around the center wavelength increases—it is a function of the laser, not the lens.
Color aberrations
In femtosecond lasers, the pulse length is linked to the spectral width of a laser pulse. As the pulse width of a laser decreases into the range of femtoseconds, the pulse spreads out in frequency. For example, a 10 ps pulse at 1064 nm has a spectral width of about 0.3 nm, resulting in essentially no pulse spreading. At the other extreme, a 50 fs pulse at 1064 nm has a spectral width of about 60 nm, yielding much broader spectral content such that the pulse contains wavelengths from 1034 to 1094 nm, resulting in a "color error" unless the lens is color-corrected.
In USP lasers, the spectral width is defined by the pulse duration as:
The constant (time-bandwidth product) depends on the actual pulse shape (see Fig. 1). For a Gaussian pulse shape, it is equal to 0.441. If equality is obtained in the above equation, then it is a transform-limited pulse, meaning that for a given spectral width, there is a lower limit for the pulse duration.
A problem most severe at longer wavelengths is that the shorter the pulse duration, the larger the spectral width of the pulse (see Fig. 2). For pulse lengths in the picosecond regime, the spectral width is around 1 nm and below, and can usually be neglected. In that case, fused silica lenses that are color-corrected monochromatically for just one wavelength can be used and only one glass material is required.Correcting color errors
Most optical imaging lenses that span the human visible spectrum such as binoculars or machine-vision imaging lenses correct for color errors by combining various glass types with different indices of refraction and different Abbe numbers. The Abbe number is a measure of the material's chromatic dispersion—its variation of the refractive index vs. wavelength.
Nanosecond and picosecond pulse lasers have a very small spectral spread on the order of a few nanometers or less, resulting in essentially no wavelengths that are out of focus, both axially in z and laterally in the x and y scan field. In that case, fused-silica lenses designed for a single wavelength and corrected monochromatically can be used. However, femtosecond USP lasers are more challenging.
To illustrate the spectral bandwidth impact on the performance of an F-Theta scan lens, the Sill telecentric F-Theta scan lens S4LFT4010/328 with 100 mm focal length used with a 10 mm (1/e2, vignetted at 1/e2) input beam and a maximum field size of 35 × 35 mm was analyzed (see Fig. 4). Unlike a normal focusing lens that has field curvature so only the center ray would be in focus on a flat field, an F-Theta scan lens is designed to be in focus over the entire image plane of the field scanned by the laser.Designed for one wavelength only at 1064 nm, this F-Theta lens shows that spot performance in the corner of the scan field (a location at which the color errors are at their maximum) results in color errors both in the transverse (scan-length direction) and propagation direction (focal-length direction). At 400 fs, the spot shape is essentially the same as for a 10 ps or longer pulse, and the lateral color error is small in respect to the spot size. The image size of the Huygens point-spread function (PSF) plot is 40 μm and the lateral color error is approximately 8 μm. But at 50 fs, the lateral color error is approximately 60 μm and the aberrations are quite obvious and extreme.
To combat this color error, Sill Optics designed the proprietary, multi-element, multi-material S4LFT7010/450 telecentric F-Theta scan lens with a focal length of 100 mm and a maximum field size of 35 × 35 mm with color correction from 1.0 to 1.1 μm for a 100-nm-wide color spectrum. For a 10 mm beam, the lens is diffraction-limited (the maximum theoretical resolution possible) with a lateral color error in the field corner below 1 μm by design, resulting in a very round nonaberrated spot.
The S4LFT7010/450 is being used in the European-founded project ADALAM (grant agreement number 637045; http://www.adalam.eu) with a goal of developing an adaptive laser micromachining system based on USP laser ablation and a novel depth-measurement sensor, together with advanced data analysis software and automated system calibration routines.
Complementary with this new F-Theta scan lens, Sill Optics has also introduced the S6ASS4803/450 3X beam expander that is color-corrected from 1.0 to 1.1 μm and diffraction-limited for a 10 mm beam (double 1/e2 diameter).
Ghost reflections
Ghost (or back) reflections occur as a portion of the laser light is reflected back from various lens elements. Most F-Theta scan lenses contain anywhere from 2 to 6 lens elements. Because lens surfaces typically reflect back about 4% of the light energy on each surface, laser lenses use antireflection coatings to transition the light from the index of refraction of the air to the refractive index of the bulk material of the lens (see Fig. 5), reducing the back reflection from each surface to about 0.2%. Although 0.2% seems like a small amount, the peak power of the ghost spot in a pulsed laser can exceed the damage threshold of the coating.If the reflection is focused into the air space between lens elements or inside the bulk material of the lens, then it is not a problem. However, if the back reflection is focused onto the surface of a previous lens element or onto a galvo scan mirror surface, the energy density can burn a spot into the coating. The solution is an optical design that avoids these focused back reflections and maintains all the other constraints (focal length, scan field size, spot size, and glass types, among others) within the optical design. Back reflections onto the galvo scan mirrors are controlled by using the correct F-Theta lens adapter ring thickness.
Günter Toesko is senior project manager, laser components at Sill Optics, Wendelstein, Germany; e-mail: [email protected]; and Christian Dehnert is applications and sales engineer at CourierTronics, Troy, NY; e-mail: [email protected].