OPHTHALMOLOGY: Broadband nonplanar AO scanning ophthalmoscope clearly images rods
Researchers at the Flaum Eye Institute and the Institute of Optics, both at the University of Rochester (Rochester, NY), have created a reflective scanning ophthalmoscope that for the first time clearly and directly images rods in the living human eye.1 The device consists of four off-axis afocal telescopes formed by pairs of spherical mirrors in a nonplanar arrangement and includes an adaptive optics (AO) deformable mirror. It uses one or more light sources (which can be broadband) in the 450–850 nm range and has many potential uses, including optical coherence tomography (OCT), hyperspectral imaging, and single- and multiphoton fluorescence. The instrument was tested on a human subject by the Rochester team and researchers from Marquette University and the Medical College of Wisconsin (both in Milwaukee, WI).2
In the eye, the light-sensitive cells known as cones are concentrated at the center of the retina in the fovea centralis; they provide high visual resolution and color vision. Rods are much more numerous than cones, are more sensitive to light, and exist mainly in locations other than the fovea. The rods, which are monochrome sensors and are used in low-light vision, have previously been difficult to image clearly, even with the use of AO.
Nonplanar arrangement cancels astigmatism
The new ophthalmoscope achieves sharper imaging due to the nonplanar folding of the optical path, which reduces both pupil- and image-plane astigmatism at the same time. The lower astigmatism at the pupil plane reduces the wavefront deviation and the beam wander due to scanning, easing the work that the AO mirror has to do. The lower image-plane astigmatism allows the ophthalmoscope to achieve diffraction-limited performance over a 3-diopter vergence range (the higher the vergence range, the greater the depth of focus; the thickness of the human retina and nerve fiber add up to about 360 µm, or about 1-diopter vergence).
To simultaneously compensate for astigmatism at both planes, the magnification of the afocal telescopes must be as close to 1:1 as possible. In the off-axis design, a larger field of view (FOV) necessitates a larger off-axis angle for the design, resulting in a poorer optical performance; thus a tradeoff must occur between maximum FOV and minimum acceptable optical performance. The resulting design is optimized for a 7.5 mm beam diameter at the eye, a 5.75º vertical FOV, and a 2.45º horizontal FOV. The researchers note that the price to pay for the instrument’s very high image quality is a large size—almost 2 m in its longest dimension; however, the length could be reduced using a different scanner and AO mirror, they add.
Light sources include an 850 nm laser diode, a 680 nm superluminescent diode (SLD) with an 8.5 nm bandwidth, and a 796 nm SLD with a 14 nm bandwidth; light from all sources was delivered to the instrument via singlemode fiber. Scanning is done with a nonresonant galvo scanner, while photomultiplier tubes are used as detectors.
Reflectance images of a human eye were taken with a 27-year-old male emmetrope (someone with excellent vision without glasses) as the subject. The sinusoidal scan data was made linear by estimating the distortion from images of a Ronchi ruling and then resampling the data. The photoreceptor images can be displayed using a linear grayscale versus image intensity (see figure), or using a logarithmic grayscale mapping, which could be more useful for OCT.
The researchers say their next step is to develop a clinical version that can be made widely available. A related task is teaching the art of interpreting AO images to guide clinical decisions about diagnosis and treatment.
REFERENCES
1. A. Dubra and Y. Sulai, Biomedical Opt. Exp., 2, 6, 1757 (June 1, 2011).
2. A. Dubra et al., Biomedical Opt. Exp., 2, 7, 1864 (July 1, 2011).
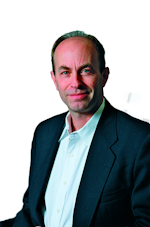
John Wallace | Senior Technical Editor (1998-2022)
John Wallace was with Laser Focus World for nearly 25 years, retiring in late June 2022. He obtained a bachelor's degree in mechanical engineering and physics at Rutgers University and a master's in optical engineering at the University of Rochester. Before becoming an editor, John worked as an engineer at RCA, Exxon, Eastman Kodak, and GCA Corporation.