PHOTONIC FRONTIERS: METAMATERIALS: Optical metamaterials seek real-world applications
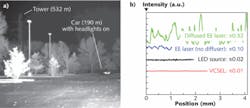
Synthetic metamaterials have excited the optical community because they promise optical properties unavailable from any conventional materials. A stunning early example was the demonstration of a negative refractive index, something long thought impossible. Optical invisibility cloaks have intrigued the popular press.
Yet despite some elegant laboratory experiments, practical questions remain. What use are invisibility cloaks if they only work for microscopic objects illuminated by monochromatic light? It seems tempting to call metamaterials “a solution looking for a problem,” like the laser was called in its early days. A new round of developments, however, is promising important practical capabilities not available from conventional optical materials, including broadband polarizing filters, near-perfect absorption, and optics for the terahertz band.
Metamaterial basics
Metamaterials are assemblies of subwavelength structural elements designed to collectively interact with electromagnetic waves. The structural building blocks act as miniature inductive-capacitive circuits that interact with the electric and magnetic fields that make up the light wave. Typically the structures are designed to interact much more strongly with magnetic fields than conventional materials. The effects are particularly strong at frequencies that have resonances with the structures.
In principle, these interactions can produce a vast range of optical properties that are unobtainable from conventional materials, such as negative refractive index. Moreover, varying the shape of the structure or the configuration of the building blocks can create gradients in optical properties that can manipulate light, a field called “transformation optics” because the structures transform the wavefront.1 It’s also possible to change the structures dynamically to vary their properties.
Although experiments have yielded impressive results, they also have revealed important practical limitations. Optical attenuation is high because many important structural elements are metals. The most impressive results have come at long wavelengths—particularly in the microwave range—because it’s much easier to fabricate subwavelength structures for those bands than for visible light. In fact, fabrication is so complex that typical experiments use only a single layer of metamaterial unit cells—few demonstrations have used truly three-dimensional structures. Yet these problems do not stop development of metamaterials to solve some particularly troublesome problems with real-world applications.
Microwave and terahertz optics
The first metamaterial experiments were in the microwave band, and developers are working on metamaterial devices including phased array antennas, says Claudio Parazzoli of Boeing Phantom Works (Seattle, WA). However, more “optical” devices are being developed for the terahertz band, where conventional optical devices are problematic and wavelengths are long enough that structures measuring tens of micrometers—easily producible by conventional semiconductor techniques—count as subwavelength.
The high absorption of metamaterials can be an attraction in the terahertz band, where natural materials generally have low absorption. An 8 µm single-layer metamaterial, for example, has been made with absorptivity of 0.7 at 1.3 THz, or an absorption coefficient of 2000 per centimeter (see Fig. 1).2 Terahertz absorbers also have been made insensitive to polarization and to angles of incidence.
A recent review paper catalogued many other terahertz metamaterial demonstrations, including quarter-wave plates, switching and modulation, and tuning of resonance behavior by moving structural elements. Developers also have reported shifting resonant frequencies as much as 20% in materials with memory effects that after 20 minutes or more return the metamaterial to its original configuration, desirable for switching and modulation experiments. “In many cases, the [metamaterial] based terahertz devices outperform their conventional counterparts, though in many cases such conventional counterparts do not exist at terahertz frequencies,” writes a team from Boston University and Boston College (Boston, MA).3
Infrared metamaterials
Metamaterial lenses also are attractive for infrared wavelengths, where conventional lenses tend to be bulky and hard to coat. “A very thin lens of negative-index materials, with very few layers, could do a lot for lidar applications,” says Steve Brueck, director of the center for high technology material at the University of New Mexico (Albuquerque, NM).Another potentially important infrared application is in high-speed switching and modulation. Response time depends on device size, which in bulk materials ranges from centimeters in periodically-poled lithium niobate to kilometers in fiber media. Semiconductor optical amplifiers can switch over shorter distances because they are active devices, but they add noise and complexity. Plasmonic switches have subpicosecond response, but modulation depth has been limited to 10% in micrometer-scale devices.
Brueck’s group and colleagues at the Los Alamos National Laboratory (Los Alamos, NM) have demonstrated 600 fs response in a submicron metamaterial layer. They used a two-dimensional “fishnet” structure, with an amorphous silicon dielectric separating two thin silver layers (see Fig. 2). Illuminating the silicon with fast green laser pulses generated electron-hole pairs, which shorted out the two metal sheets, making the structure act like a single metal-like grid with different resonances until the electrons and holes dissipated and the structure returned to its normal resonance. The group measured modulation depth of about 70%, suggesting the system might be suitable for high-speed switching.4
Circular polarization
Another attraction of metamaterials is their ability to produce effects such as strong circular polarization, which can be difficult with conventional bulk optics. Early demonstrations showed strong chirality and negative phase velocity in the microwave and terahertz range, but the effects were limited to narrow bandwidths.Martin Wegener’s group at the University of Karlsruhe (Karlsruhe, Germany) overcame this problem by stretching the widely used split-ring resonator structure into a helix. A single-cycle helix produced circular polarization of infrared light transmitted along the axis of the helix. When the group extended the helix to two complete cycles, they produced broadband circular polarization, transmitting most right-hand circularly polarized light and little left-hand circularly polarized light across an octave from 3.5 to 7.5 µm (see Fig. 3).5
The group made the metal helixes by focusing femtosecond laser pulses onto tight spots in a photopolymer resist, scanning the pulses to create a 3D structure in the polymer, then depositing a thin gold film over the polymer helixes. The coated coils then can be used for infrared sensing of chiral molecules.1 “We have been working on improving its performance, and companies have expressed interest,” says Wegener.
His group is also exploring the use of magnetic control to achieve perfect impedance matching—and thus perfect absorption—of incoming light by the metamaterial. So far, the structures have converted the absorbed energy into heat, but converting the energy into other forms could make it useful for sensing. Harry Atwater’s group at the California Institute of Technology (Pasadena, CA) is trying to make perfect absorbers to improve the performance of solar cells.
Active metamaterials: gain to counteract loss
Seeking to overcome the troublesome losses in metamaterials, some developers are inserting gain media into the structures. Last year, Vladimir Shalaev’s group at Purdue University (West Lafayette, IN) showed that placing a gain medium such as rhodamine 6G dye into zones where local electromagnetic field intensities were particularly high could achieve enough gain to offset losses.6
Other ways to add gain to metamaterials also are being studied, including quantum dots and optical parametric amplification. “Loss compensation in metamaterials is a crucial step toward their practical applications,” writes Allan Boardman of the University of Salford (Greater Manchester, England) with nine co-authors in a review of active and tunable metamaterials.7 Gain and tunability have become hot areas, as evidenced by the review’s 130 references.
Looking forward
The concept of metamaterials has tremendous potential, but making that potential a reality requires overcoming serious practical issues such as device fabrication and loss. Finding successful near-term applications will help sustain long-term support for broader development. Last year, Nikolay Zheludev at the University of Southampton (Southampton, England) called chirality, negative refractive index, and strong magnetic response the ripest fruits of the “metamaterial tree of knowledge.”8 He also pointed to other fruits still growing and ripening, such as slow light, switching, and amplification.
Zheludev wrote that better methods of fabrication are crucial. “New techniques will have to achieve perfection of nanostructures at close to the molecular level, and at low cost.” He wants the new approaches “to occupy a position between chemical processes controlled by self-organizing forces on the truly molecular level and the less accurate, top-down methods which can build metamaterials to almost any blueprint.” That’s a huge challenge, but the rewards will be even larger.
REFERENCES
1. M. Wegener and S. Linden, “Shaping Optical Space with Metamaterials,” Physics Today, 63, 32 (Oct. 2010).
2. H. Tao et al., “A metamaterial absorber for the terahertz regime: Design, fabrication and characterization,” Opt. Exp., 16, 7181–7188 (May 2008).
3. H. Tao et al., “Recent Progress in Electromagnetic Metamaterial Devices for Terahertz Applications,” IEEE J. Selected Topics in Quant. Electron., 17, 92–101 (January/February 2011).
4. K.M. Dani et al, “Ultrafast nonlinear optical spectroscopy of a dual-band negative index metamaterial all-optical switching device,” Opt. Exp., 19, 5, 3973 (Feb. 28, 2011).
5. J. Gansel et al., “Gold helix photonic metamaterial as broadband circular polarizer,” Science, 325, 1513–1515 (Sept. 18, 2009).
6. S. Xiao et al., “Loss-free and active optical negative-index metamaterials,” Nature, 466, 735–738 (Aug. 5 2010).
7. A. Boardman et al., “Active and tunable metamaterials,” Laser & Photon. Rev., 5, 2, 287–307 (2011); doi: 10.1002/lpor.201000012.
8. N.I. Zheludev, “The road ahead for metamaterials,” Science, 328, 582–583 (Apr. 30, 2010).
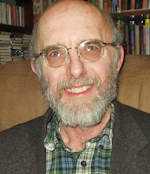
Jeff Hecht | Contributing Editor
Jeff Hecht is a regular contributing editor to Laser Focus World and has been covering the laser industry for 35 years. A prolific book author, Jeff's published works include “Understanding Fiber Optics,” “Understanding Lasers,” “The Laser Guidebook,” and “Beam Weapons: The Next Arms Race.” He also has written books on the histories of lasers and fiber optics, including “City of Light: The Story of Fiber Optics,” and “Beam: The Race to Make the Laser.” Find out more at jeffhecht.com.