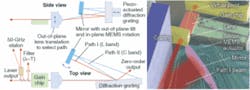
Grating-tuned external-cavity semiconductor lasers have served for many years as convenient, widely tunable laboratory laser sources and have found applications as diverse as spectroscopy, biomedical testing, and optical component testing. They have not been commonly used outside the laboratory, however, because of limitations related to size, cost, and reliability.
One spectral region where such lasers are useful is the telecommunications window around 1550 nm. This eye-safe spectral region is suitable for fiberoptic sensing and molecular overtone spectroscopy. It is particularly attractive because of the existence of robust, telecommunications-derived optical components, including the nontunable distributed-feedback (DFB) lasers often used to implement simplified field versions of techniques developed with tunable lasers.
Recently, Telcordia-qualified tunable lasers have become available, including MEMS (microelectromechanical systems)-based devices that use a grating-tuned external-cavity laser technology analogous to that used in laboratory-tunable lasers.1 Tunability in optical networks is used to eliminate expensive multiwavelength DFB inventories and to allow optical routing to be used in reconfigurable optical networks. For this application, the lasers are optimized to lock to a specific grid of frequencies and to stay locked for many years without interruption, through a range of environmental perturbations. There are challenges, however, in adapting these rigidly controlled devices for spectroscopy and sensing, in which the desired frequencies are not on a simple grid and the laser needs to sweep repetitively over a continuous range of frequencies without mode hops. This is a difficult problem for some grid-tuned designs, but in the case of MEMS-based devices, the required modifications are minor and do not affect device reliability.
In a MEMS-tuned external-cavity diode laser, the gain chip output is collimated by the lens, diffracted by the grating, and retroreflected to the gain chip (see Fig. 1). Using an indium gallium arsenide phosphide (InGaAsP) gain chip with a 10-THz gain bandwidth and appropriate gratings, 20-mW output powers in the C or L bands can be coupled to polarization-maintaining fiber with a polarization-extinction ratio better than 20 dB. The devices have relative intensity noise (RIN) less than -155 dB/Hz from 1 MHz to 13 GHz and spontaneous-emission background of less than -50dBc/nm. The instantaneous linewidth is less than 20 kHz, with low-frequency actuator jitter that broadens the 25-µs average linewidth to 2 MHz.
Frequency is tuned by rotating the mirror with a MEMS actuator fabricated by deep reactive-ion etching from single-crystal silicon. A flexural structure with a thickness of approximately 100 m gives good out-of-plane stiffness, allowing a separately fabricated silicon mirror to be bonded to the actuator and rotated through a 6.2° angle about a virtual pivot. Single-crystal silicon does not exhibit material fatigue, and more than 50 billion device cycles have been observed without failure. The filter and 50-GHz etalon give absolute frequency references that are combined with a phase signal based on the zero-order output signal for servo-controlled grid tuning of the laser.2 The laser components are assembled on a ceramic substrate, bonded to a thermoelectric cooler, and sealed in a hermetic butterfly package. The laser and its drive electronics fit in a 50 × 70 × 13-mm module.
Path I or II is selected using an out-of-plane lens adjustment to bring the desired reflection into alignment with the gain medium. A fixed lens can select either path, or a linear MEMS actuator that translates the lens can allow active switching between paths. The multiple paths expand the tuning range: with the components normally used for an L-band laser, path I scans from 1565 to 1608 nm and path II (with only slightly lower efficiency) scans from 1514 to 1574 nm. A third path (two diffractions at the grating and a second normal incidence reflection at the mirror) operates at lower efficiency between 1488 and 1542 nm.3, 4
These paths appear as peaks at 54, 68, and 80 V in the MEMS lens voltage scan (see Fig. 2). The path I and path II spectra show 60-dB suppression of the nonselected frequency. Path II is longer, so it has smaller cavity-mode spacing, but the additional diffraction at the grating gives a corresponding increase in spectral resolution so the side-mode suppression ratio (SMSR) is similar for both bands.A laser with a MEMS-tuned lens was calibrated for path II grid-tuned operation at 50 GHz and, under harsh Telcordia-like environmental conditions, gave performance indistinguishable from a conventional 20-mW C-band telecommunications laser. Channel switching times of less than 10 ms could be obtained within a single band. Because control of the lens was not optimized, the times required to switch between bands was several times longer. Path II has the feature that double-passing the mirror increases the relative path-length change associated with a given mirror rotation. Designing a MEMS virtual pivot actuator for path I mode-hop-free tuning is difficult because the actuator performance is generally optimized with a pivot point closer to the actuator than would be required to obtain the mirror translation needed for continuous tuning. Using path II in combination with adjustment of the piezo-grating position actuator gives enough cavity-length change to achieve mode-hop-free tuning over the entire path II tuning range.
For scanning, the MEMS actuator voltage is cycled to give an approximately linear wavelength ramp, while a slower grating servo adjusts the cavity length to avoid mode hops, based on the zero-order output signal.2 During a 0.4-Hz wavelength scan from 1520 to 1570 nm (C band plus 5-nm guard bands), the scan rate determined from the 50-GHz etalon signal stayed in the 60 ± 25-nm/s range in both scan directions across the entire scan (see Fig. 3). Similar full-range scans are routinely achieved at 2 Hz, and scan rates of several hundred Hz over shorter tuning ranges are also possible.MEMS-tuned external-cavity semiconductor lasers have been adapted for spectroscopic and sensing applications by exploiting the multiple configurations that exist in the resonator. Use of a MEMS-actuated adjustable lens allows switching between multiple resonator configurations, providing an effective way of increasing the tuning range. Proper choice of configuration allows mode-hop-free-tuning without requiring impractically large travel from the MEMS actuators. The resulting lasers are useful in a variety of sensing and spectroscopy applications.
REFERENCES
- J. D. Berger and D. Anthon, Optics and Photonics News 14(3), 42 (March 2003).
- D. Anthon et al., Optical Fiber Comm. Conf. and Exhib., paper MF61 (OFC 2003).
- D. Anthon et al., Conf. on Lasers and Electro-Optics, paper CWL2 (CLEO 2004).
- D. Anthon et al., Optical Fiber Comm. Conf. and Exhib., paper WL2 (OFC 2004).
DOUG ANTHON is director of optics, JILL D. BERGER is manager of optical design, and SUBRATA DUTTA is an optical engineer at iolon, 1870 Lundy Ave., San Jose, CA 95131; e-mail: [email protected].