PHOTONIC FRONTIERS: METAMATERIALS AND TRANSFORMATION OPTICS: Newest metamaterials promise customized optical properties
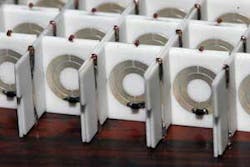
One of the hottest fields in optical research is undergoing an exciting transformation. Metamaterials research has given birth to a new field called transformation optics that promises to greatly extend the potential of assembling synthetic optical materials from subwavelength structures.
The first generation of metamaterials was built from identical and uniformly spaced subwavelength structures. Interactions of the electric and magnetic fields of light waves with the subwavelength modules produced effects that are impossible in natural materials, such as negative refractive indices. Their development offered the exciting potential to design entirely new types of optical materials.
Now developers have extended the concept to transformation optics, in which the modules and/or the spacing are not uniform throughout the material. Tailoring the complex nanostructure transforms the wavefront passing through it, enabling entirely new operations such as optical cloaking. The new field of transformation optics is “a new paradigm for the science of light,” says Vladimir Shalaev of Purdue University (West Lafayette, IN).
Metamaterial basics
Metamaterials were first demonstrated for microwaves, and extended to visible light by nanofabrication technology. Their optical properties are shaped by the interactions of arrays of subwavelength structures with the electric and magnetic fields that make up light waves (see Fig. 1). Because their internal structures differ from conventional materials, their optical properties can be very different.
The demonstration of negative refractive index was the real eye-opener. Optical engineers normally think of refractive index n as the ratio of the speed of light in vacuum to that in a material, but the underlying physics means the index actually is the square root of the product of electrical permittivity e and magnetic permeability µ.Both quantities are defined as 1 in a vacuum, so n = 1 for vacuum.
Dielectrics such as glass have positive permittivity and permeability, so their refractive indices are positive. Conductors have negative permittivity and positive permeability at visible wavelengths, so these opaque materials have imaginary refractive indices. No natural materials have negative values of both quantities, but a decade ago John Pendry of Imperial College (London, England) showed that metamaterials could be made with both permittivity and permeability negative at selected wavelengths.
Since then negative-index superlenses have been demonstrated at optical wavelengths. The first used only single layers of modules, but last year Jason Valentine and Xiang Zhang at the University of California (Berkeley, CA) stacked layers to make the first three-dimensional metamaterials.1 But that was only a starting point.
Transformation optics and cloaking
Negative-index metamaterials are uniform, containing many identical and evenly spaced modules, so their optical properties are uniform as well. Changing the shape and spacing of internal structures within a metamaterial in an arbitrary way opens the door to transforming light in other ways. It’s a very powerful concept. Citing the late Arthur C. Clarke’s observation that, “any sufficiently advanced technology is indistinguishable from magic,” Ulf Leonhardt at the University of St. Andrews (St. Andrews, Scotland) and David R. Smith of Duke University (Durham, NC) wrote last year that transformation optics were “the first practical demonstrations of ‘pure and applied magic.’ ”2
The specific bit of magic that caught their eyes, and those of the popular press, is invisibility cloaking by deflecting light around an object in the middle of an area so the wavefront that reaches an observer seems to have come straight through the cloaked object, as if it wasn’t there (see Fig. 2). Like negative-index materials, cloaking was first seen for microwaves and then demonstrated for light.Broadband cloaking is possible in other cases. This spring, two groups described broadband “carpet cloaks” that could hide a bump under a rug. Optically, previous cloak designs compress the hidden object into a single point or line from the viewer’s standpoint, wrote Valentine, Jensen Li, and Thomas Zentgraf of Zhang’s group at Berkeley, but “the carpet cloak compresses an object in only one direction into a conducting sheet.”3 That change in the shape of the wavefront doesn’t require singularities or drastic changes in properties through the material, allowing them to avoid resonance and use low-loss dielectric materials to achieve much broader bandwidth than is possible with conductors.
Valentine and colleagues drilled an array of 110 nm holes into a 250-nm-thick silicon slab optical waveguide. The researchers spaced the holes regularly except in a rectangular cloaking region, where the holes were arranged in a two-dimensional pattern designed to cloak a bump on the edge of the slab waveguide (see Fig. 3). When they directed a laser beam at a bump “under” the cloak, they saw a single Gaussian peak in the reflected light, as they would from a flat edge. Illuminating an uncloaked bump revealed multiple peaks, as expected for reflections from a bump. They observed the cloaking effect from 1400 to 1800 nm.
Lucas Gabrielli and colleges in Michal Lipson’s group at Cornell University (Ithaca, NY) demonstrated a similar dielectric carpet cloak, in which 50 nm silicon posts were embedded in a silica layer. Distribution of the silicon posts determined the optical transformation of the wavefront. The group also reported that its cloak concealed a bump on the edge of the planar waveguide across a 400 nm range in the 1550 nm region. Without the cloak, light reflected from the bump at an angle included a dark shadow-like zone, but with the cloak light reflection from the bump area was as smooth as if the area had been flat.4
Optical black holes
The power of transformation optics comes from the ability of a nonuniform metamaterial to warp the path of light arbitrarily, like a gravitational field can warp space-time. Zhang is working to extend the analogy so light propagation through metamaterials can be used in laboratory studies of such cosmic phenomena as black holes and strange attractors in the laboratory.Zhang envisions devices he calls “continuous-index photon traps,” which could serve as “perfect” optical cavities that absorb light but don’t radiate across a broad range of wavelengths. One possibility is to combine air and the high-index semiconductor indium gallium arsenide phosphide (InGaAsP) to form a “photonic black hole,” which would attract light in the same way a gravitational black hole attracts matter.5 Zhang says transformation optics could design devices “that can mimic the periodic, quasi-periodic, and chaotic motions observed in celestial objects that have been subjected to complex gravitational fields” (see Fig. 4). One use could be to simulate the chaotic long-term orbits of planets and asteroids. “Unlike astronomers, we will not have to wait 100 years to get experimental results,” Zhang says.
Separately and nearly simultaneously, Evgenii E. Narimanova and Alexander V. Kildishev of Purdue proposed using transformation optics to make omnidirectional broadband light absorbers, or “optical black holes.” But they weren’t thinking of research applications. They envision practical applications including photovoltaics, solar-energy harvesting, and optoelectronic detectors.6
A similarly intriguing application of metamaterials is an omnidirectional retroreflector, recently demonstrated with microwaves by Leonhardt’s group. Like conventional retroreflectors made of multiple mirrors, the devices would reflect all incident light back in the direction from which it came, not just the ray incident on a reflective sphere at a right angle. With conventional optics, such a device would require a dielectric singularity with an infinite refractive index, but Leonhardt’s group used transformational optics to convert that singularity into a mere topological defect in a metamaterial.7
State of a fast-moving art
Although the idea of transformation optics has been around a while, key experiments have been reported within the past few months. As this article was about to go to press, Science published the first report of frequency-tunable “memory metamaterials,” in which frequency response can be both tuned and preserved.8 The tuning, demonstrated in Dimitri Basov’s lab at the University of California at San Diego (La Jolla, CA), is based on hysteresis in vanadium oxide structures in the metamaterial, and could lead to many other devices.
With transformation optics, says Shalaev, “you are limited only by your imagination because you really can do anything with light.” His excitement at his group’s recent demonstration of visible cloaking an area 100 wavelengths wide illustrates the state of the field.9 That’s only 50 µm, the width of a human hair, and a long way from hiding Harry Potter or even a covert microphone. But it’s an important step toward a bright future, and could lead even further. “You could make a cloak for light, you could make a cloak for electron wave functions, and you could make electron black holes,” he predicts. Stay tuned.
REFERENCES
- J. Valentine et al., Nature 455, p. 376 (Sept. 18, 2008) doi:10.1038/nature07247
- U. Leonhardt and D. R. Smith, 2008 Focus on Cloaking and Transformation Optics New J. Phys. 10, p. 115019; doi: 10.1088/1367-2630/10/11/115019
- J. Valentine et al., Nature Materials (2009) doi:10.1038/nmat2461
- L. Gabrielli et al., Nature Photonics 3, p. 461 (Aug. 2009) doi:10.1038/nphoton.2009.117
- D. Genov et al., Nature Physics (July 20, 2009) (doi:10.1038/Nphys1338)
- E. E. Narimanova and Alexander V. Kildishev, Applied Phys. Lett. 95, 041106 (2009).
- Y. G. Ma et al., Nature Materials 28 (June 2009) doi:10:1038/nmat2489
- T. Driscoll et al., ScienceExpress 20 (August 2009) (doi:10.1126/science.11765580)
- I. Smolyaninov et al., Phys. Rev. Lett. 102, 213901 (May 29, 2009).
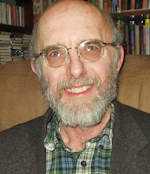
Jeff Hecht | Contributing Editor
Jeff Hecht is a regular contributing editor to Laser Focus World and has been covering the laser industry for 35 years. A prolific book author, Jeff's published works include “Understanding Fiber Optics,” “Understanding Lasers,” “The Laser Guidebook,” and “Beam Weapons: The Next Arms Race.” He also has written books on the histories of lasers and fiber optics, including “City of Light: The Story of Fiber Optics,” and “Beam: The Race to Make the Laser.” Find out more at jeffhecht.com.