Silicon Photonics: Standard wire-bonding technique doubles waveguide Ge detector bandwidth
Some forms of active silicon photonic circuits benefit from the use of germanium (Ge) as a photodetector material, creating a hybrid system. These Ge waveguide-coupled photodetectors can take the form of either vertical or lateral p-i-n junction structures. While lateral structures have reached a 3 dB bandwidth of 120 GHz, the easier-to-make vertical structures have had difficulty exceeding a 3 dB 30 GHz bandwidth using many but very complicated techniques.
Now, researchers from the Huazhong University of Science and Technology (Wuhan, China) and Huawei Technologies (Shenzhen, China) have come up with a straightforward way of boosting the speed of conventional vertical p-i-n junction Ge detectors from 30 to 60 GHz.1 The technique involves using standard wire-bonding technology to introduce gold wires into the detector's discrete ground electrodes.
The so-called RC parasitic parameter (R and C refer to the photodetector equivalent circuit's resistance and capacitance components, respectively) places a limit on how fast a detector can operate. However, introducing an inductor (L) into the detector's RC circuit to create a so-called RLC circuit can help high-speed operation because the introduced inductance counteracts part of the influence of the capacitance.
Avoiding the creation of any complicated on-chip inductors, the research group simply wire-bonds gold wires to the Ge detector, creating an "off-chip" inductor (although the wires actually reside at the Ge detector itself). To do this, they change the detector's single conventional integral electrode to three separate parts (see figure), leaving the actual photodetection section unchanged. Then, they introduce two gold wires to connect the two discrete ground electrodes—the section of wire forms the inductor, with its inductance value depending on the size and length of the wire.
For example, a detector can be made using a gold wire about 450 μm long and with a 25 μm diameter, which provides an inductance and resistance of 1 nH/mm and 2 Ω/mm, respectively, over a wide frequency range. For this device, calculations show the doubling of the detector's bandwidth from 30 to 60 GHz.
Experiment verifies concept
An experimental Ge photodetector was fabricated on a silicon wafer using a 0.18 μm CMOS process at the Institute of Microelectronics in Singapore. First, a single-mode channel waveguide with a 500 nm width was fabricated in the silicon, along with a grating coupler etched to a depth of 70 nm for vertically coupling light into the detector. Next, doped layers of Ge totaling about 700 nm were deposited and then etched, and metals were deposited to form the electrodes. Post-fabrication bonding of two pieces of gold wire totaling about 450 μm in length completed the device.
Bonding of the wires slightly decreased the detector's responsivity from 1 to 0.85 A/W at 1550 nm under a -3 V bias voltage, attributed to the resistance of the wires. At a bias voltage of -0.5 V, the bonded wires actually slightly reduced the dark current from 64 to 61 nA.
The detector's frequency response was measured by coupling light at a 1550 nm wavelength to the device through a cleaved fiber at a power of about 5 dBm. While some jitter was seen in the measurements at higher frequencies because of bandwidth limitations in the electrical cable and connections used in the measurement, a 3 dB response out past 60 GHz was seen.
The researchers note that because wire-bonding technology is a standard part of a Ge waveguide detector's construction anyway, their method adds no extra fabrication process. In addition, the bonded wire can easily be removed and a new wire of same or different length and diameter rebonded.
REFERENCE
1. G. Chen et al., Opt. Express (2015); doi:10.1364/OE.23.025700.
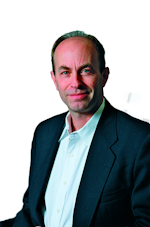
John Wallace | Senior Technical Editor (1998-2022)
John Wallace was with Laser Focus World for nearly 25 years, retiring in late June 2022. He obtained a bachelor's degree in mechanical engineering and physics at Rutgers University and a master's in optical engineering at the University of Rochester. Before becoming an editor, John worked as an engineer at RCA, Exxon, Eastman Kodak, and GCA Corporation.