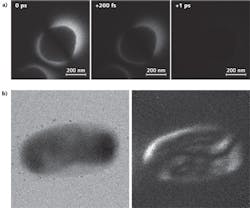
Transmission electron microscopy (TEM) continues to be a powerful imaging tool at the nanometer scale and below. But a revolutionary technique developed in late 2009 by the California Institute of Technology (Caltech; Pasadena, CA) called photon-induced near-field electron microscopy (PINEM) marries electron and photon interactions at the nanoscale to enable femtosecond time-scale images of microscopic processes—truly adding the fourth dimension of time to nanoscale imaging.¹ Less than half a year later, the PINEM technique is being applied to the life sciences and producing 4D, label-free images of ultrafast processes occurring within biological structures.²
Photons boost electron contrast
In electron microscopy, the passage of electrons through a specimen to be imaged results in the kinetic energy either being conserved (elastic process) or in a transfer of energy to the specimen (inelastic process). These no-loss elastic or low-loss inelastic electron scatterings are used to form an image. For specimens with a high atomic number, contrast is strong for the static image (time averaged over seconds). However, because biological specimens and tissues have a low atomic number, standard TEM produces weak-contrast images and scientists must resort to ultrathin sectioning and staining techniques or complex energy filtering methods.
The demonstration of the laser-assisted surface photoelectric effect and other photon-electron interactions such as plasmonics laid the groundwork for Caltech's development of the PINEM technique. When a femtosecond laser pulse and an ultrashort electron packet are overlapped in space and time at a nanostructure in situ, a unique region of energy gain is observed—electrons absorb photon energy. Both the absorption and emission of light by the electrons produce peaks in the energy spectrum located at integer multiples of the photon energy. For PINEM, the electrons that have absorbed photons are selected by energy filtering and an image showing those gain events over time can be formed. Because only electrons traveling near the specimen absorb photons, nanoscale contrast is enhanced for any sample regardless of atomic number.
Imaging biological samples over time
To demonstrate the feasibility of producing time-resolved images of biological processes, E. coli cells were imaged using the PINEM technique (see figure). At 0 fs, maximum spatiotemporal overlap of the laser pulse and electron packet occurs and the E. coli structure is clearly visible. After only 200 fs, the PINEM contrast is considerably weaker, and is nearly nonexistent after just 1 ps, indicating the utility of the method for imaging on ultrashort time scales. The PINEM images offer enhanced contrast compared to TEM for both the outer (membranes) and inner (ribosomes, DNA material) of the E. coli cell; apparently, the photon-electron interaction improves contrast significantly compared to electron-only interactions.
In addition to time-resolved images, tomographic or 3D images are possible with PINEM by taking images of the specimen at different tilt angles. Varying the polarization of the femtosecond laser pulse also enhances contrast (and provides additional spatial information) for a sample under test. Additional variants including laser wavelength could reveal even more information as the PINEM technique is explored and refined.
"The extension of PINEM to systems having properties much different than metals and semiconductors, such as cells and proteins, demonstrates the versatility of the technique," says Caltech postdoc David J. Flannigan. "Add to this the ability to resolve dynamics in situ on the femtosecond timescale and it becomes clear that potential applications are limited only by one's imagination."
REFERENCES
1. B. Barwick et al., Nature 462, 902–906 (Dec. 17, 2009).
2. D.J. Flannigan et al., Proc. Natl. Acad. Sci. (PNAS) 107, 22, 9933–9937 (Jun. 1, 2010)
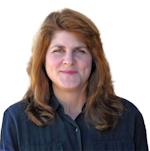
Gail Overton | Senior Editor (2004-2020)
Gail has more than 30 years of engineering, marketing, product management, and editorial experience in the photonics and optical communications industry. Before joining the staff at Laser Focus World in 2004, she held many product management and product marketing roles in the fiber-optics industry, most notably at Hughes (El Segundo, CA), GTE Labs (Waltham, MA), Corning (Corning, NY), Photon Kinetics (Beaverton, OR), and Newport Corporation (Irvine, CA). During her marketing career, Gail published articles in WDM Solutions and Sensors magazine and traveled internationally to conduct product and sales training. Gail received her BS degree in physics, with an emphasis in optics, from San Diego State University in San Diego, CA in May 1986.