LASER OPTICS: Deformable thermal-lens-correcting mirror has single actuator
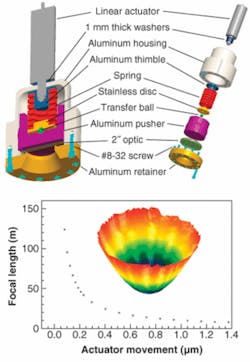
To counter thermal lensing in high-energy-laser rod amplifiers, researchers at Sandia National Laboratories (Albuquerque, NM) have developed a deformable mirror that does one thing, and does it well. Incorporating only a single actuator, the mirror corrects for a wavefront error that has a parabolic dependence on radius-the classic thermal-lensing effect. The simple, rugged actuator mechanism applies a radially symmetric moment to a circular flat mirror to deform it in the right way (see figure).1
The Nd:phosphate glass laser used by the Sandia researchers contains a three-stage optical parametric chirped-pulse-amplifier (OPCPA) front end, two glass-rod amplifiers with 16 and 25 mm diameters, and ten glass-slab amplifiers with 41 × 41 cm clear apertures. The laser produces pulses with diameters of 12 cm and energies of up to 225 J; these pulses are then compressed to 600 fs duration with a vacuum-grating compressor at a 65% efficiency. With peak powers ranging up to 100 TW, the pulses enter a 1.5-m-diameter target chamber for high-energy-physics experiments. The Sandia laser operates at a very low repetition rate.
To measure the shape of the wavefront error, which the researchers term spherical aberration, the researchers probed the optical system with a low-power continuous-wave laser (contrary to conventional optical terminology, in which “spherical aberration” refers to a fourth-power wavefront aberration, the term in this case refers to the spherical wavefront that is created when a flat wavefront traverses a thermal lens caused by a parabolic temperature profile). The instantaneous thermal lensing during shot time was probed with the OPCPA output beam.
Negative instantaneous thermal lens
During the shot, a strong negative thermal lens is formed, which then persists for 5 and 21 seconds in the 16- and 25-mm-diameter rods, respectively. As the heat from the shot suffuses through the rod and the water-cooling draws the heat away, the thermal lens becomes positive, slowly decaying with time constants of 27 and 91 seconds respectively for the two amplifier rods. Although the positive lens disappears between shots if the wait is long enough, the negative lens is instantaneous (and proportional to the magnitude of the shot) and must be compensated for before the shot-the rationale for the deformable mirror.
In the mirror assembly, a 50.8-mm-diameter, 3-mm-thick mirror (30 mm clear aperture) is held between a stationary ring of 32.5 mm diameter and a pusher ring of 47.2 mm diameter constrained by a cylindrical bore. A screw-drive linear actuator presses on a spring that converts actuator displacement to force on the mirror.
Two mirror assemblies were fabricated, one with a polished and the other with an unpolished pusher surface; in interferometric tests with the first four Zernike terms subtracted out, the polished pusher produced an rms (root-mean-square) wavefront error ranging from 0.005 to 0.008 waves depending on the force, while the unpolished pusher produced about twice as much error (focal lengths from 100 m down to 5 m were measured). In actual laser tests, the focal spot of an uncompensated rod shot had a Strehl ratio of 0.03; compensation by the deformable mirror under the same conditions raised the Strehl ratio to 0.21 (a decrease in spot size from 38 to 9.4 µm in x and 24 to 12.1 µm in y).
Researcher Jens Schwarz notes that the quickly and readily adjustable deformable mirror will have multiple applications in remote sensing, laser-induced-breakdown spectroscopy, lidar, laser machining, multiphoton microscopy, and control of laser-filament formation. Sandia is interested in making the mirror technology (which is patent-pending) available to companies to further develop and mature the technology into a market-ready product. While the Sandia laser operates in single-shot mode, the high-repetition-rate (for example, 10 Hz) laser amplifiers used in many other places tend to form a constant positive thermal lens; this problem can be compensated with the same deformable-mirror concept by creating a convex mirror, notes Schwarz.2
REFERENCE
1. J. Schwarz et al., Optics Express 14(23) 13 (November 2006).
2. J. Schwarz et al., Applied Physics B: Lasers and Optics 82, 275 (2006).
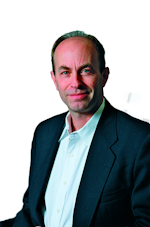
John Wallace | Senior Technical Editor (1998-2022)
John Wallace was with Laser Focus World for nearly 25 years, retiring in late June 2022. He obtained a bachelor's degree in mechanical engineering and physics at Rutgers University and a master's in optical engineering at the University of Rochester. Before becoming an editor, John worked as an engineer at RCA, Exxon, Eastman Kodak, and GCA Corporation.