OPTICS FOR SOLAR ENERGY: Holographic planar concentrator increases solar-panel efficiency
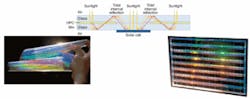
Worldwide solar-energy output has grown dramatically in recent years, particularly in Europe, China, and the U.S. The total output from all solar installations worldwide, however, still remains around 7 GW, only a tiny fraction of the world’s energy requirement.1 High material and manufacturing costs, low solar-module efficiency, and a worldwide shortage of refined silicon all have limited the scale of solar-power development required to effectively compete against coal and liquid fossil fuels.
A number of approaches are being explored to improve the cost per kilowatt of solar power, primarily by improving the efficiency of the solar-collecting cells that make up the solar modules, or by concentrating greater amounts of solar energy onto the cells.
The holographic planar concentrator (HPC) is an approach that achieves both of these goals. An HPC is built up from several layers of film composed of gelatin on polyethylene terephthalate. In each film, holographic optical elements are imprinted using diode-pumped solid-state lasers. The holographic stack diffracts wavelengths that are usable by the solar cells while allowing unusable wavelengths to pass through unabsorbed. The usable energy is guided via total internal reflection at the glass/air interface to strings of solar cells, resulting in up to a threefold concentration of energy per unit area of photovoltaic material.
Mono- and bifacial designs
A monofacial module (that is, one that collects solar energy from the front only) incorporates HPC film and strips of photovoltaic (PV) solar cells (see Fig. 1). Because of the HPC film, this module uses 50% less PV material than a traditional fully populated module. The reduction in silicon lowers the module’s material cost and results in manufacturing savings through reduced assembly and processing requirements.
A second important benefit of the HPC is that it collects indirect and diffuse light throughout the day and through clear and cloudy conditions. The holographic films collect light over a large angular range. Light entering at angles within this range is diffracted to the PV cell. This “passive tracking” characteristic allows the HPC to produce electrical power through more hours of the day and during overcast conditions.
The third advantage of the HPC is that it diffracts only the wavelengths of sunlight that can be converted to electricity by the PV cells (approximately 400 to 1100 nm). The performance of PV cells tends to drop significantly as temperature increases. In an HPC-based module, infrared radiation, which would be converted to heat rather than output energy in conventional modules, passes through the HPC film and is not absorbed. The HPC’s ability to pass unusable wavelengths means that the solar cells remain close to their ideal operating temperature without mechanical cooling, even at 3× concentration.
Solar modules with HPC concentrators are currently being developed that achieve higher efficiency ratings than the component PV cells within them. For example, a module can incorporate bifacial PV cells (see Fig. 2). The cells, which collect energy from both the front and back surfaces, are placed between strips of HPC film, which also collect from the front and back and from a wide range of incident angles.
In a bifacial design, the cells receive greater irradiance than in a conventional monofacial design. In the example configuration, the PV cells see 2.4 to 3.4 suns (1 sun = 1000 W/m2) of total irradiance over long periods of the day.
The bifacial HPC configuration uses 72% less silicon than a fully populated module. This can also be viewed as a 300% increase in watts per gram of silicon, an important consideration in light of the current worldwide shortage of refined silicon. This reduction in silicon and other improvements combine to lower the cost of a solar module to below a dollar per watt.Efficiency gains
The efficiency of an HPC-equipped module remains high for lower-light conditions, meaning that an HPC module will generate significantly higher yield in real-world lighting conditions (see Fig. 3). In addition, an HPC module tends to perform better than a conventional module as temperature increases, both because of the HPC’s ability to pass infrared wavelengths and because heat can be dumped into a larger volume of material than in a fully populated module.
Other module savings are achieved by closely packing the silicon strings and using fewer, more-efficient interconnections. The HPC-equipped module can also be somewhat smaller than a PV module of comparable peak power rating, resulting in further cost savings in framing and support structures.
One last advantage of the HPC module is that, because of its inherent passive tracking characteristics, it can be mounted in a variety of locations and orientations, including commercial white roofs, flat and inclined roofs, windows, vertical walls, and so on. Passive tracking increases the irradiance to the solar cells even when they are mounted in a less than optimal orientation.Measuring HPC efficiency
The purpose of the holographic element is to diffract sunlight with a broad range of incident angles and wavelengths to the PV cell regions. The hologram should also efficiently diffract scattered and diffuse light to the PV cell regions. The diffraction efficiency is defined as light diffracted by the hologram relative to light incident on the hologram. The diffraction efficiency of a volume grating can be close to 100% in practice even when the incident beam angles and wavelength change (see Fig. 4).
Incident sun angles change as a result of the daily and seasonal paths of the sun. The solar-PV-cell spectrum is determined by the product of the air mass 1.5 global solar spectrum (a standard test condition for PV cells) and the responsivity of the PV cell. For a silicon PV cell, the responsivity ranges from about 400 to 1100 nm.
The “efficiency” of a solar cell is often quoted as its power output at “full sun.” This value is measured by flashing 1000 W/m2 (one sun) onto a test cell held at 25°C. While this method is effective for comparing conventional cells, it is not as useful for comparing modules, for several reasons. First, the full-sun condition is rarely seen in actual use. For most locations, the great majority of available light occurs from 0.2 to 0.6 suns due to steep incident angles, cloudiness, haziness, and so on. Second, modules that take advantage of low-incidence and reflected light increase the irradiance level seen by cells to a level above one sun. And third, temperature significantly impacts efficiency, and of course the temperature increase is most apparent as the irradiance level approaches full sun. The temperature coefficient is not represented in the single efficiency number.
A more effective way to compare novel modules, such as HPC-based designs, to conventional modules is to measure the energy yield per gram of silicon in the module. Assuming a PV-cell thickness of 240 µm, an HPC module of equivalent size and power output requires three grams of silicon per watt of output power, versus eight grams per watt in a conventional panel. If the newer cells with a 180 µm thickness are incorporated, along with improvements to the HPC design, that number improves to nearly a watt of output for every gram of silicon.
Incorporating HPC into module manufacturing
HPC production is a roll-to-roll process that can be readily incorporated into the manufacture of modules based on crystalline or amorphous PV cells, thin films, or other solar-cell technologies.
An additional production step is required for HPC-based modules: standard PV cells must be diced into 12- to 25-mm-wide strips that can be placed between the holographic film strips. A low-cost laser-cutting technique has been developed that provides clean cuts that give the cells excellent mechanical strength. The cells also have slightly (approximately 5%) better efficiency than the original wafer as well.
The ease of incorporating HPC into module designs should lead to rapid adoption of the new technology. As solar installations continue to increase, economies of scale will drive the price down even further. Reducing silicon requirements by two-thirds will be an important part of that strategy for the foreseeable future.
REFERENCE
- M. Rogol et al., Solar Annual 2008, Photon Consulting.
Mike Zecchino | Technical Communications, 4D Technology
Mike Zecchino is in technical communications at 4D Technology, Tucson, AZ.
Glenn Rosenberg | Chief Technology Officer, Prism Solar Technologies
Glenn Rosenberg is the chief technology officer of Prism Solar Technologies (Lake Katrine, NY) and the inventor of holographic planar concentrator (HPC) technology.
Raymond K. Kostuk | Professor, University of Arizona
Raymond K. Kostuk is a professor in the Electrical and Computer Engineering Department and the College of Optical Sciences at the University of Arizona, Tucson, AZ.