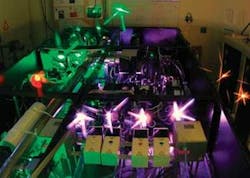
By adding a booster amplifier to their 50 TW Hercules laser, researchers at the University of Michigan (UM; Ann Arbor, MI) have pushed its peak power to 300 TW—the highest ever achieved for a repetitively pulsed laser. The team also focused the 30 fs pulses to an intensity of 2 × 1022 W/cm2, the highest ever recorded from a laser. “I don’t know of another place in the universe that would have this intensity of light,” said Karl Krusheinck, one of the researchers and coauthor of a report in Optics Express.1
The Lawrence Livermore National Laboratory (Livermore, CA) produced the first petawatt pulses a decade ago with chirped-pulse amplification, and other laboratories have followed (see www.laserfocusworld.com/articles/266389). But those systems are limited to single shots because they use glass amplifiers, which dissipate waste heat slowly. Hercules uses Ti:sapphire amplifiers, with much better heat dissipation that allows a 0.1 Hz repetition rate imposed by the glass pump lasers, says coauthor Victor Yanovsky, who added that diode pumping of solid-state lasers might yield 100 J pulses at 10 Hz (see figure).
In 2004 Yanovsky generated peak powers of 45 TW from Hercules, and focused pulses to a then-record intensity of 1022 W/cm2.2 After a regenerative Ti:sapphire amplifier boosted seed pulses to 40 mJ, the output was directed to a cryogenically cooled four-pass amplifier followed by a final two-pulse amplifier. A deformable mirror corrected wavefront distortion, and an f/0.6 off-axis parabolic mirror focused the beam to a 0.8 µm spot, with peak intensity of 1022 W/cm2.However, amplified spontaneous emission from the system posed a problem. Amplifiers normally produce a prepulse of amplified spontaneous emission lasting around a nanosecond, which Yanovsky says is “forever” on the timescale of a femtosecond pulse. That prepulse is only about 10-6 or 10-7 the power of the femtosecond pulse, but when the system optics focus the main pulse to an intensity of 1022 W/cm2 the prepulse is powerful enough to destroy the target before the main pulse reaches it. To overcome that problem, Yanovsky two years ago used a technique called cross-polarized wave generation to reduce amplified spontaneous emission to a level only 10-11 of the femtosecond pulse.3
Pumping up the pulse power
Now his group has added a two-pass Ti:sapphire booster amplifier that pumps up the femtosecond pulse power by a factor of six at 0.1 Hz, generating 17 J pulses that, after compression, have peak power of 300 TW and a pulse width of 30 fs at a nominal center wavelength of 810 nm. Focusing those pulses onto a target with a f/1.0 parabolic mirror gives peak intensity of 2 × 1022 W/cm2. Yanovsky explained that they chose not to use the f/0.6 lens because its focal length is so short that something is likely to obstruct the output before it reaches the target. If the technique can be extended to the shorter-focus lens, power density should reach 5 × 1022 W/cm2.
That intensity is close to the level of 1023 to 1024 W/cm2 where interesting new physics is expected. Those power densities should produce radiation reaction effects that affect electron motion, offering a test of electrodynamic models that treat electrons as points. A more practical application of such intensities would be to accelerate protons or ions for cancer therapy. Penetrating the body requires electron energies of about 200 MeV, which now can only be achieved with expensive particle accelerators. Current laser acceleration is limited to about 50 MeV—too low to make ions penetrate the body, but higher laser intensities could boost electron energies to the required 200 MeV range.
It won’t be easy to crank power up to that range. “We are pretty much close to the limit on the focal spot,” says Yanovsky; there is no room to go to mirrors faster than f/0.6 at 800 nm. Pulses might be squeezed down to 10 fs, which could yield up to a factor of three increase in peak power, but that’s about the limit. Building a bigger laser in principle could yield as much pulse energy as you could afford, but reaching an energy sufficient to generate 100 pW would cost at least $100 million.
The ultimate pulse intensity would be about 1029 W/cm2.
REFERENCES
1. V. Yanovsky et al., Optics Express 16, 2109 (Feb. 4, 2008)
2. S.-W. Bahk et al., Optics Lett. 29, 2837 (Dec. 15, 2004)
3. V. Chvykov et al., Optics Lett. 31, 1456 (May 15, 2006).
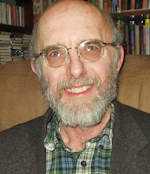
Jeff Hecht | Contributing Editor
Jeff Hecht is a regular contributing editor to Laser Focus World and has been covering the laser industry for 35 years. A prolific book author, Jeff's published works include “Understanding Fiber Optics,” “Understanding Lasers,” “The Laser Guidebook,” and “Beam Weapons: The Next Arms Race.” He also has written books on the histories of lasers and fiber optics, including “City of Light: The Story of Fiber Optics,” and “Beam: The Race to Make the Laser.” Find out more at jeffhecht.com.