New design work by researchers from Aalto University, the University of Eastern Finland, Karlsruhe Institute of Technology, and Harbin Engineering University—based on theoretical models and electromagnetic simulations—takes us a step closer to realistic photonic time crystals.
This same team created two-dimensional (2D) photonic time crystal metasurfaces, a.k.a. a time-based version of conventional optical materials, back in 2023.
Related Article
“Photonic time crystals represent a unique class of optical materials,” says Xuchen Wang, who was a postdoctoral researcher at Karlsruhe Institute of Technology in Germany, but is now a full professor at Harbin Engineering University in China. “They have bizarre electromagnetic properties—remaining uniform in space while exhibiting a periodic oscillation in time.”
This distinctive quality creates “momentum bandgaps,” or unusual states where light pauses inside the crystal while its intensity grows exponentially over time (see Fig. 1). To appreciate the peculiarity of light’s interaction within a photonic time crystal, imagine light traversing a medium that switches from air to water quadrillionths of times per second—a remarkable phenomenon that challenges our conventional understanding of optics.
Significance of the bandgap within momentum space
In this latest work, the team discovered a method to expand the momentum bandgap via resonant structures. The momentum bandgap is crucial because it’s the underlying mechanism that facilitates light amplification—and expanding the momentum bandgap allows a broader range of light momenta to be amplified, which leads to more effective light amplification.
“Typically, increasing the size of the momentum bandgap requires enhancing the modulation depth,” Wang points out. “Most current research about photonic time crystals assumes a high modulation depth to create a large momentum bandgap. But such high modulation power, as suggested in most theoretical models, is often impractical in experiment and could potentially damage the material.”
The bandgap in momentum space implies that for momenta within this bandgap, the eigenfrequency—frequency at which an oscillatory system oscillates without external excitation—is complex. This complex eigenfrequency indicates that light will be amplified exponentially over time according to Maxwell’s equations.
A big challenge for the team was figuring out how to achieve a wide momentum bandgap to effectively amplify the light momenta—and it’s precisely what their latest work focused on solving.
They figured out how to achieve a substantial momentum bandgap with a moderate modulation depth that’s feasible using current technology. “Using a time-varying resonant material can significantly expand the momentum bandgap without increasing the modulation depth,” says Wang. “This resonance can either stem from the material’s intrinsic properties or be induced by restructuring nonresonant materials into resonant metasurfaces.”
Importantly, an optical resonant metasurface can enhance the momentum bandgap by as much as 350x compared to nonresonant structures. “This breakthrough is a pivotal advancement for the realization of photonic time crystals for optical applications,” says Wang.
Cool design work
One of the key challenges of the team’s latest work was creating a highly resonant structure with very flat dispersion curves (see Fig. 2).
“Under time modulation, these flat dispersion curves fold and repel to form a wide momentum bandgap,” explains Wang. “Following this idea, we used an array of dielectric spheres characterized by strong Mie resonances and structured low-loss silicon into two-dimensional (2D) arrays of resonant spheres.”
This configuration allows Wang and colleagues to effectively use the Mie resonances to significantly enlarge the momentum bandgap under time modulation (making it approximately 350x larger than typically achieved within nonresonant regimes). “Our results aren’t merely theoretical—this marks a crucial advancement toward the practical implementation of photonic time crystals at optical frequencies,” he says.
To analyze the band structure of time-varying bulk media, the team’s approach “combines the dynamics of the Lorentz dispersion model with the plane wave expansion method,” says Wang. “For our metasurface study, we use an equivalent circuit model to analyze the band structure of photonic time crystals, followed by COMSOL full-wave simulations to validate the concept. To model optical metasurfaces, we use the T-matrix method, based on multipole expansions, to calculate the band structure with high accuracy.”
One potential application for the team’s photonic time crystals is nanosensing. “Imagine we want to detect the presence of a small particle, such as a virus, pollutant, or biomarker for diseases like cancer,” he says. “When excited, a particle emits a tiny amount of light at a specific wavelength, and a photonic time crystal can capture and automatically amplify it—which would enable more efficient detection with existing equipment.”
Other potential applications include communications, imaging, and sensing, thanks to the team’s work to create the building blocks for compact and faster lasers, sensors, and other optical devices.
4D photonic time crystals ahead?
Why shoot for 4D time crystals? Conventional 3D optical materials are passive systems that don’t allow wave amplification because it would violate the principle of energy conservation.
“By incorporating time as an additional degree of engineering freedom, light can be amplified because energy can be effectively drawn from the modulation source and pumped into the material system,” says Wang. “This capability is crucial for enhancing light-matter interactions and overcoming optical losses within optical materials.”
What’s the next step? “We’ll focus on the experimental demonstration of photonic time crystals at optical frequencies based on the ideas of our latest work,” says Wang.
FURTHER READING
X. Wang et al., Nat. Photon. (2024); https://doi.org/10.1038/s41566-024-01563-3.
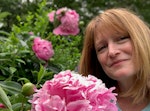
Sally Cole Johnson | Editor in Chief
Sally Cole Johnson, Laser Focus World’s editor in chief, is a science and technology journalist who specializes in physics and semiconductors.