In an impressive step away from relying solely upon global positioning system (GPS) satellites for navigation, scientists at Sandia National Laboratories developed a multichannel silicon photonic modulator chip. It’s part of a laser system designed for cold-atom interferometry—a form of quantum sensing to measure acceleration and angular velocity—and was created at the National Security Photonics Center (see video).
Their device is an ultraprecise quantum inertial measurement unit (IMU) option wherever GPS is unavailable or for situations when satellite signals are intentionally denied or jammed.
This is an evolving national security risk within war zones, and motion sensors are advancing at a rapid rate now—dramatically shrinking in size and cost, while increasing in capability. Until recently, this type of sensor would have filled a large truck and cost significantly more. Shrinking bulky, expensive sensor components to fit on silicon photonic chips also drives down their cost.
And, thanks to the principles of quantum mechanics, quantum IMUs now offer unparalleled accuracy measuring acceleration and angular velocity—which enables ultraprecise navigation.
“The necessity of compact and rugged laser systems in configuring various quantum sensors inspires my work within this field,” says Jongmin Lee, the Sandia scientist who led this work. “In particular, cold-atom interferometry relies on sophisticated and often bulky laser systems that limit the size, weight, and power consumption of quantum sensors. The challenge of miniaturizing and ruggedizing these laser systems is crucial for developing deployable quantum gravity and inertial sensors based on atom interferometry.”
Chipscale photonic modulator design
An atom interferometer sensor system typically fills a small room—they’re not known for being small—and a complete quantum IMU requires six atom interferometers.
Lee and his team set out to find ways to reduce the size, weight, and power needs of atom interferometers. They ended up creating a tiny photonic chip (8 mm × 8 mm), including four single-sideband modulators with custom features, which serves as the heart of a laser system on a microchip—and replaces a refrigerator-sized conventional laser system.
Four of these modulators are necessary to shift the frequency of a single laser to perform different functions. But a big downside of modulators is they tend to generate unwanted echoes, a.k.a. sidebands, which must be mitigated. To outmaneuver it, Lee and colleagues designed a suppressed-carrier, single-sideband modulator that reduces these sidebands by 47.8 decibels (also applicable to light intensity) and provides a nearly 100,000-fold drop.
Importantly, they can be fabricated using the same process as computer chips. The team can make hundreds of their modulators on one 8-inch wafer, or more on a 12-inch wafer, which significantly reduces their cost.
PIC laser system architecture
The Sandia scientists’ photonic integrated circuit (PIC) laser system architecture begins with a single telecom laser—because it has a longer lifetime and narrower linewidth than 780-nm distributed Bragg reflector lasers or distributed-feedback lasers—locked to an atomic transition, which creates multiple coherent light beams through single-sideband modulators.
The architecture of this laser system “is divided into three functional blocks: light modulation, optical amplification, and frequency doubling—from 1560 nm to 780 nm—for rubidium atoms,” explains Lee.
Cold-atom interferometry requires cold-atom generation, state-selective atom detection, and a light-pulse sequence for matter-wave interference. “This process involves several key components: cooling, repumping, depumping, detection, and two Raman beams,” Lee says. “The amplitude and frequency of each beam must be precisely controlled in a timed sequence. Most of the complex and costly light modulation tasks can be managed using a multichannel silicon photonic single-sideband modulator chip, which includes custom features.”
The size, weight, power consumption, and cost of conventional tabletop laser systems “limit the deployability of quantum sensors,” Lee says. “Quantum inertial measurement units, multi-axis gravimeters, and multi-axis gravity gradiometers, in particular, require multiple laser systems.”
Sandia’s PIC laser system architecture can miniaturize and ruggedize the laser systems for cold-atom interferometry by leveraging silicon photonics that rely on the same complementary metal-oxide semiconductor (CMOS) fabrication process extensively used for microchips. This approach “enables mass production with a high yield rate, which ultimately reduces the cost of these quantum sensors and accelerates the development of field-deployable quantum sensors,” Lee says.
Remaining challenges ahead include achieving low-loss optical packaging between a fiber array and a photonic chip, as well as performing low-loss photonic wire bonding between various integrated photonic chips. “We’re also focused on demonstrating high-performance quantum sensors using a fully functional PIC laser system,” says Lee. “Beyond this, we’re exploring the optimal methods to operate quantum gravity and inertial sensors under conditions of vibrations and high dynamics.”
Most surprising aspect of this work? “This work is a result of a multidisciplinary collaboration between the physics team and the photonics team,” Lee points out. “Understanding both fields to design, implement, and test the PIC laser system for atom interferometry was quite challenging. One of the most rewarding moments was successfully demonstrating a proof-of-concept quantum sensor in the laboratory—it was an exhilarating experience that validated our efforts and highlighted the potential of our approach.”
Quantum sensors and laser-based sensing ahead
As far as applications, the multichannel silicon photonic single-sideband modulator chip developed at Sandia can be widely applied to various quantum sensors and laser-based sensing technologies. “We’re continuing to advance this technology within the given time and budget constraints, and we expect it to be ready for real-world applications within the next five to 10 years,” says Lee.
Beyond navigation, Lee sees potential for their optical components, including the modulator, for LiDAR, quantum computing, and optical communications applications.
The Sandia team is now exploring various PIC technologies, including silicon photonics, III-V photonics, nonlinear optics, and MEMS-based photonics. “Through multidisciplinary collaborations, we aim to identify the best PIC solutions for specific quantum applications,” Lee adds. “Also, we plan to provide quantum sensing testbeds through the Quantum New Mexico Institute for academia and industry—to enable other researchers to test their PIC laser systems with our testbeds.”
FURTHER READING
A. Kodigala et al., Sci. Adv., 10, eade4454 (2024); https://doi.org/10.1126/sciadv.ade4454.
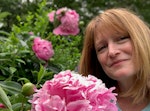
Sally Cole Johnson | Editor in Chief
Sally Cole Johnson, Laser Focus World’s editor in chief, is a science and technology journalist who specializes in physics and semiconductors. She wrote for the American Institute of Physics for more than 15 years, complexity for the Santa Fe Institute, and theoretical physics and neuroscience for the Kavli Foundation.