Quantum electrodynamics theory unveils precise ‘shape’ of a single photon
In what led to a breakthrough for quantum physics and photonics, Angela Demetriadou, a professor of theoretical nanophotonics, and Benjamin Yuen, a research fellow in theoretical quantum nanophotonics, set out to answer a fundamental question: How are photons really emitted by atoms and molecules, and what effect do their geometry and the optical properties of the environment have on them?
Physicists have searched for an answer to this question for a long time but, until now, it could only be answered with simplified models. The team’s work enables precisely defining how a photon interacts with matter and other elements of its environment (see video below).
“I recall a discussion about this with my Ph.D. supervisor Professor Ed Hinds of Imperial College, London, more than a decade ago, and it’s lingered over me ever since,” says Yuen. “When I first met with Angela, I realized that with our combined expertise we were onto something.”
Recently, there’s an increased interest in coupling experimentally quantum emitters—whether fluorescent molecules, quantum dots, etc.—to open nanophotonic systems. “But most of the theoretical descriptions we had available to us so far to describe the light-matter interactions were either phenomenological or making assumptions that were limiting our understanding of the processes involved and some experimental findings,” says Demetriadou.
So they created a rigorous theoretical description for these interactions “to gain greater understanding of these light-matter interactions to see how they can be used for quantum technology applications with new nanophotonic systems,” Demetriadou says. “Ben did a remarkable job in developing the theory, and we got a lot more out of it than we expected.”
Quantum dynamics
It started by trying to “describe the quantum dynamics of an atom, molecule, or any other simple quantum emitter with the resonant modes of a photonic nanostructure,” explains Yuen. “Classically, such photonic resonances are well known but their quantum dynamics was a problem we felt we needed to solve.”
In quantum terms, things appear less concrete. There is a quantum state or ‘wavefunction’ that instead describes the system. It accounts for all possible paths along which the system evolves and how these interfere with one another.
“For the electromagnetic field in isolation, the classical and quantum behavior can be remarkably similar because Maxwell’s equations embrace interference and free photons don’t interact, but when an atom or other quantum emitter is involved it can be drastically different,” Yuen says. “In some ways the atom is like a conduit for the energy to excite many more light modes, while it can also enter into a cycle of emission and reabsorption that cause the photon’s existence to pulse on and off.” (See video below.)
Evolution of the photon as it exchanges energy back and forth with the atom on the nanosphere. (Video credit: https://doi.org/10.1103/physrevlett.133.203604)
This complexity has meant most models consider a much simpler version of these kinds of systems. While these models are very effective for describing macroscopic situations like an atom within a high finesse cavity, “nanophotonic systems aren’t so clear-cut: They have many broadband and overlapping resonances, radiate efficiently to the far field, and there is no clear boundary between ‘inside’ and ‘outside’ like you have with a cavity,” Yuen points out.
The team’s first task was “to combine the machinery of quantum field theory with all the details provided by classical electromagnetism to produce a complete and exact theory. Once we did this, we then had to figure out a way to solve the quantum equations of motion using finite computational resources,” Yuen adds.
Main takeaways
First, visualizations are useful to physicists and everyone else. The image the team made may be aesthetically pleasing, but together with the rest of their theory, it shines a spotlight on what’s really going on within a somewhat esoteric process.
Second, Demetriadou and Yuen welcome the new era of quantum and photonic engineering with open arms. Studies like theirs provide the tools to harness quantum mechanics in new ways that when combined with photonic engineering can change the way we communicate securely, detect pathogens, or harvest energy from light, to name merely a few.
“Our work is applicable to other research areas, such as controlling photochemical and photocatalytic reactions with nanophotonic devices, which involves the quantum interaction of molecules with nanophotonic environments,” says Demetriadou.
Finally, they hope to inspire a fresh, more global interpretation for quantum mechanics. “By embracing the complexity of the environment, we showed an excited atom decays just as radioactive atoms that trigger the demise of Schrödinger’s cat,” Yuen says. “But in our case, we didn’t need the controversial ‘wavefunction collapse’ caused by an observer. By building better quantum models, we may just find that the randomness of quantum mechanics is instead replaced by complexity and chaos.”
As with most groundbreaking work, they encountered cool surprises along the way. “Quite amazingly, several details just started to drop out of our theory, such as exactly how light propagates to the far field, and exactly what the shape of the photons’ intensity distribution is expected to be,” Yuen says. “This was surprising because we didn’t explicitly specify these characteristics—they appeared because we incorporated a complete set of solutions to Maxwell’s equations into our quantum model. It was also very reassuring that we were on the right path.”
Yuen was also quite astonished when he realized their theory describes all the dynamics of an “open quantum system,” which is incredibly difficult to achieve without making significant approximations.
Quantum theory challenges
Demetriadou and Yuen faced two clear challenges to overcome with their theory. First: Write down an accurate quantum theory of a seemingly lossy photonic system that radiates energy to the far field. And once they had this quantum theory, they had to make it tractable without needing to solve the energy exchange between the atom and each one of the infinite light modes present within the continuous frequency spectrum.
The first challenge they faced was to avoid the many pitfalls encountered when considering quantum systems that lose energy through radiation. “To navigate around these we instead considered the entire universe as one closed but infinitely large system,” says Yuen. “But by doing so, we gave ourselves the second challenge: A need to solve a continuum of possible interactions between the atom and each frequency mode of light within a continuous spectrum.”
They found a way to transform the continuum of possible interactions into a discrete set of them. “We used a branch of mathematics known as complex analysis to transform the problem from a continuous set of real valued frequencies into a discrete set of complex valued ones,” explains Yuen. “This provided an enormous simplification of the problem and allowed us to precisely represent the problem using just a few hundred of these ‘complex’ light modes.”
Ultimate goal now? “To build a numerical tool to solve the quantum electrodynamics for any nanophotonic system, regardless of its material properties or shape,” says Yuen. “Ideally we’d like to build a complete computational package that allows engineers to quantize and solve any nanophotonic system in a user-friendly way. Our work provides a big step in this direction, which ultimately will unlock a new generation of quantum photonic engineering.”
FURTHER READING
B. Yuen and A. Demetriadou, Phys. Rev. Lett., 133, 203604 (Nov. 14, 2024); https://doi.org/10.1103/physrevlett.133.203604.
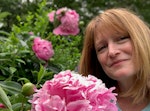
Sally Cole Johnson | Editor in Chief
Sally Cole Johnson, Laser Focus World’s editor in chief, is a science and technology journalist who specializes in physics and semiconductors. She wrote for the American Institute of Physics for more than 15 years, complexity for the Santa Fe Institute, and theoretical physics and neuroscience for the Kavli Foundation.