Optical Cooling: All-optical cryogenic cooling of sensors eliminates vibration
Mechanical cryogenic coolers are used to reduce the signal-to-noise ratio of some optical detectors, particularly those working in the infrared (IR) spectral region (such coolers have many other uses in precision science as well). Unlike simply using a dewar filled with liquid nitrogen, which evaporates relatively quickly to provide cryogenic cooling, a mechanical cooler can keep an optical sensor in operation for long periods of time, enabling long-term remote use—for example, on a satellite.
However, mechanical cryogenic coolers have a problem: they produce vibration. For some sensitive optical detection schemes, vibration is a serious hindrance. (And thermoelectric coolers, which do not produce vibration, cannot by themselves cool devices from room temperature to true cryogenic temperatures.)
All-optical cooling made practical
A solution to this problem has now been developed by researchers at Los Alamos National Laboratory (Los Alamos, NM) and the University of New Mexico (Albuquerque, NM) that is based upon the exotic phenomenon of all-optical cooling, and which has been put into functional form as a cryocooler for a mercury cadmium telluride (HgCdTe) IR sensor in a working Fourier-transform infrared (FTIR) spectrometer.1 The experimental setup keeps the HgCdTe sensor cooled to 135 K.
The most-efficient form of optical cooling developed so far relies on laser-exciting a ytterbium-doped fluoride crystal such as YLiF4:Yb3+, causing fluorescence at a wavelength shorter than the excitation laser. As a result, the crystal experiences a loss of energy, cooling it. Mansoor Sheik-Bahae, the leader of the research group, and his colleagues have taken this phenomenon and put it into a practical form using elegant mechanical and thermal design, mounting the crystal, the sensor, and the cold finger and connecting them in a vacuum using aerogel (which is a very poor heat conductor) as the mount (see figure).
“The key component that enabled us to cool an ‘arbitrary’ load was the design and fabrication of a thermal link that sheds >99.9% of the fluorescence from the cooling crystal away from the cold finger while withstanding frequent thermal cycling,” Sheik-Bahae says. “This was achieved by a delicately shaped undoped YLF crystal that was fusion-bonded to the Yb:YLF cooling crystal. Other important factors involved enhanced coupling of laser light to the cooling crystal, which was achieved by a use of an astigmatic Herriott cell, in addition to various thermal-load-mitigation techniques.”
As for potential uses, Sheik-Bahae believes that any cryogenic application requiring a temperature of >80 K for which vibrations and microphonic noise play a detrimental role will enormously benefit from this technology—for example, focal-plane IR sensors and cryomicroscopy. “More specifically, an example of the most immediate and promising application will involve the integration of our next-generation optical cryocoolers with the NIST [National Institute of Standards and Technology] silicon reference cavity that is used to stabilize a laser to tens of millihertz linewidth,” Sheik-Bahae says. “I am particularly excited about this application, as it can allow remarkable clocks to be accessible for numerous high-precision metrology applications without needing a large footprint and cumbersome liquid-based cryocooling.”
In the short term (within one to two years), Sheik-Bahae’s group hopes to have systems that can approach watt-level heat lift in a compact and rugged architecture. Longer-term improvements include increasing the efficiency via a combination of fluorescence recycling and the use of other rare-earth-doped crystals (for example, Tm3+) as the cooling material.
“Obviously, pushing the temperatures down towards 70 K and even lower is always on our agenda,” he says. “Understanding the role of impurities, and the ability to grow extremely high-purity crystals in a reliable and consistent procedure, will be of utmost importance in achieving these goals. In collaboration with Markus Hehlen at Los Alamos National Lab, and Mauro Tonelli at University of Pisa (Italy), we are also pursuing a systematic crystal-growth approach to understand the role of transition-metal impurities that cause parasitic heating in the cooling crystals.”
Commercial promise
“We are currently partnering with Thermo-Dynamic Films (TDF, LLC), a local startup in Albuquerque (headed by Richard Epstein), which is handling the commercialization aspects,” Sheik-Bahae says. “We are also closely working with AC Materials (a crystal-growth company in Florida), whose expertise in growing high-quality cooling-grade crystals has been instrumental. All I can add is that the most costly component of an optical cryocooler is currently the laser. The good news is that, only in the last five years, the cost (dollar/watt) of such Yb-fiber lasers has decreased nearly sixfold. In short, the commercialization prospect is very promising.”
REFERENCE
1. M. P. Hehlen et al., Light Sci. Appl., 7, 15 (2018); https://doi.org/10.1038/s41377-018-0028-7.
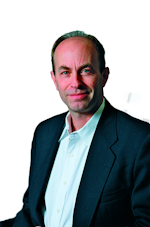
John Wallace | Senior Technical Editor (1998-2022)
John Wallace was with Laser Focus World for nearly 25 years, retiring in late June 2022. He obtained a bachelor's degree in mechanical engineering and physics at Rutgers University and a master's in optical engineering at the University of Rochester. Before becoming an editor, John worked as an engineer at RCA, Exxon, Eastman Kodak, and GCA Corporation.