Fluorescence Imaging: Fluorescence lifetime imaging measures temperature of individual cells
Every parent knows the diagnostic value of placing a hand on a child’s forehead: Measuring temperature provides quick insight into health. Researchers are now extending temperature measurement to single cells, hoping to leverage small-scale measurement into detailed information about an organism’s physiological state.
Measuring temperature on such a fine scale obviously requires a microscopic or submicroscopic temperature probe. A research team led by Professor Angel Martí of Rice University (Houston, TX) has developed such a probe. The molecular thermometer’s fluorescence lifetime changes with temperature.1 To take the temperature of individual cells, dye is introduced into culture and taken up by cells. A fluorescence lifetime imaging microscope (FLIM) then images temperature variations across the culture.
Building a molecular thermometer
Martí’s team started with the common boron-dipyrromethene (BODIPY) biocompatible fluorescent dye—a non-water-soluble molecule whose fluorescence characteristics are relatively unaffected by pH. Previous researchers had already demonstrated the ability to produce BODIPY constructs with additional chemical functional groups, called “moieties”—in this case, molecular rings attached to a single location on the BODIPY frame. Some of those moieties introduced rotational modes that affect BODIPY fluorescent properties. For a given moiety, this creates a molecule with well-defined fluorescence characteristics: absorption and emission wavelengths and fluorescence lifetime, for example. These characteristics change with temperature, but also with other physical parameters, including viscosity.
To create a probe sensitive only to temperature, Martí’s group attached a polyethylene glycol (PEG) ring to a location on the BODIPY frame, then added a couple extra methyl groups. The methyl groups inhibit free rotation, creating instead an oscillatory mode in the resulting PEG-BODIPY dye. In water, the probe has a peak excitation wavelength of 498 nm and an emission wavelength peak at 511 nm, with a quantum yield in the neighborhood of 0.2, making the probe compatible with standard fluorescence imaging tools.
At 25°C, the fluorescence lifetime is 2.7 ns. At higher temperatures, the molecule oscillates with higher energy, which opens up additional nonradiative transitions to the ground state, reducing the lifetime. At 50°C, the lifetime is 1.7 ns. The accuracy of the lifetime measurement leads to a temperature measurement resolution of 0.4°C over the biologically relevant range.
Just as importantly, the researchers found that, above a threshold value of about 41 centipoise (cP), fluorescence lifetime is independent of viscosity. This is somewhat unexpected, as unrestrained rotational modes change with viscosity. Somehow, restricting the motion to oscillatory modes nearly eliminates that dependence. Martí believes this is due to the creation of cavities at the interface between the oscillating dye and the fluid. Above the threshold, cavities form, essentially decoupling the motion of the molecule from the surrounding fluid.
Taking a cell’s temperature
Just as a parent, thermometer in hand, must find a safe way to place the probe in an ear or mouth, the researchers had to safely get their dye into the cells of interest. The choice of a PEG group makes the dye water-soluble and membrane-permeable. So, after determining an exposure limit, the team incubated cells with the PEG-BODIPY dye. Both cancerous and nonmalignant cells take up the dye. The dye is taken up differently by each cell type, concentrating in separate regions with distinct microenvironments.
Dye concentration may be affected by a variety of processes, which is why the researchers use (concentration-independent) lifetime measurements rather than relative intensity measurements. Although there may be other components, a biexponential fit to the fluorescence decay with a calculated single average lifetime is sufficient to indicate local temperature. The lifetime is, however, affected by the polarity of the surrounding fluid medium, so this method requires calibration for each cell type.
Right now, says Martí, “the main use of this probe would be for cellular temperature.” For example, it could be used to investigate heat shock response, metabolism, and hyperthermia therapies on both tumor and nonmalignant cells. “Tissue is more complex,” he adds. “We would need to use BODIPY probes with red shifted excitations.” Also, in a current development focus for the team, “we would have to encapsulate them in order to completely decouple their fluorescence from different environments in tissue.” Martí notes that there are several distinct approaches to measuring temperature, each with drawbacks and advantages. “So far, there is not a silver bullet.”
REFERENCE
1. M. Ogle et al., J. Phys. Chem. B, 123, 34, 7282-7289 (2019).
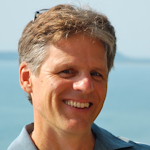
Richard Gaughan | Contributing Writer, BioOptics World
Richard Gaughan is the Owner of Mountain Optical Systems and a contributing writer for BioOptics World.