U.S. Naval Research Lab explores cold-atom quantum inertial sensors for navigation
Navigation precision is, as you can imagine, essential for military operations. Since global positioning systems (GPS) are increasingly attacked via jamming or spoofing, there’s a push to combine inertial measurement units (IMUs) as backup for GPS. IMUs rely on gyroscopes and accelerometers to track the position and orientation of objects based on a known starting point, orientation, and velocity. You can’t jam or spoof it and, unlike GPS, it also works underground/underwater or if satellites are unavailable.
Quantum inertial navigation shows promise to improve navigational system accuracy by orders of magnitude, and researchers at the U.S. Naval Research Lab (NRL) built a patent-pending three-dimensional (3D)-cooled atom beam interferometer derived from a patterned cold and continuous beam of atoms to explore atom-interferometry-based inertial measurement systems to reduce drift in navigation systems (see video).
“Our work is inspired by the Navy’s need for better inertial navigators, which help keep track of our position without an external position reference,” says Jonathan Kwolek, a research physicist working with the interferometers for NRL’s Quantum Optics Section.
Several different existing technologies are currently deployed within the fleet to perform inertial navigation, but NRL is always exploring new avenues to solve old problems. “The use of quantum-mechanical sensors as inertial navigators enables potential advantages over traditional technologies in some of the key performance metrics important for navigation,” says Kwolek.
Until now, “high-performance cold-atom quantum inertial sensors have largely been confined to laboratory demonstrations,” says Adam Black, who leads the NRL Quantum Optics Section. “We’re excited for NRL’s new sensor architecture to start making a real difference in the deployability of this promising technology.”
Cold-atom quantum inertial sensors
Cold-atom quantum inertial sensor systems exploit the quantum mechanical wave nature of matter to produce quantum interference to measure accelerations and rotations. Interferometers precisely measure displacements, refractive index changes, and surface topologies—and the systems are ideal for the U.S. Navy’s aircraft, tactical and strategic missiles, spacecraft, submarines, and ships.
Performing atomic inertial measurements vs. a classical measurement “gives different error dependencies,” Kwolek says. He predicts they will provide better long-term noise behavior and accuracy than current leading technologies. Translation: Keep your location fix longer and get the bonus of improved operational flexibility.
How do cold-atom quantum inertial sensors work? “Our cold-atom inertial sensors use many of the same technologies as other cold-atom atomic physics experiments,” explains Kwolek. “We use continuous lasers tuned near an atomic resonance to cool and spatially control the movement of atoms inside a vacuum chamber.”
Much of the laser system involves “shifting and stabilizing frequencies using acousto- and electro-optic modulators,” Kwolek explains. “These lasers are then routed through fiber optics to custom-designed optics mounts surrounding the vacuum chamber.”
The primary novelty of the NRL researchers’ work is how they generate cold atoms. Unlike many existing systems today, they generate a beam of both cold and continuous atoms in a way that mitigates issues like scattered light. “We’re working toward the combined benefits of two different interferometer architectures,” Kwolek adds.
Their interferometer shows promising characteristics such as high measurement contrast and low noise, and is better equipped to handle variations within the sensor’s environment—and these all improve the ability to continue to operate within GPS-denied environments.
One tradeoff to operating an atomic interferometer, Kwolek points out, is that enhanced sensitivity comes at the cost of dynamic range. But they’re working on a fix that may involve co-sensor implementation or alternative cold-atom techniques.
For perspective, errors in location estimation directly impact position and today’s commercially available IMUs can navigate with an error accumulation of ~1 nautical mile during 360 hours of operation. NRL’s working to extend that time so navigational drift won’t affect a mission’s duration.
Coolest aspect of this work? “Definitely the atoms,” Kwolek says. “The techniques we use to cool atoms were developed in the 1970s and 1980s, and the work was impactful enough that the inventors were awarded a Nobel Prize in the late 1990s. Building upon these techniques, our experiment achieves atomic temperatures of 15 microkelvin—almost a million times colder than the coldest place in the universe!”
Despite working within this field for more than 10 years, the fact this is possible to do still hasn’t lost its novelty for Kwolek.
One of the most difficult aspects of the experiment that remains an ongoing challenge “is setting and maintaining alignment of the lasers addressing the atom interferometer,” Kwolek says. “These laser beams have extremely stringent requirements on angular alignment, spacing, and size—and these quantities also need to be stabilized during the duration of operation. We continue to explore different methodologies and technologies to accomplish this goal.”
Wide range of applications on horizon
While this NRL project aims to directly address the need for better inertial navigators, their atomic beam source generation technique has myriad other potential applications—ranging from different sensing modalities, such as magnetic or electric field sensing, to applications within the world of timing. Kwolek and colleagues plan to explore the possibilities enabled by their patented cold-beam-generation architecture.
“As we move forward with our research, we’d like to see how the technology works in a real-world scenario,” says Kwolek. “We plan to test the interferometer on moving platforms—with a test on a ship on the open ocean. Beyond this, we’re exploring other directions within the realm of more basic research to improve our interferometer’s operation.”
FURTHER READING
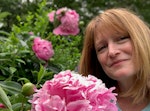
Sally Cole Johnson | Editor in Chief
Sally Cole Johnson, Laser Focus World’s editor in chief, is a science and technology journalist who specializes in physics and semiconductors. She wrote for the American Institute of Physics for more than 15 years, complexity for the Santa Fe Institute, and theoretical physics and neuroscience for the Kavli Foundation.