OPTICAL-FREQUENCY MEASUREMENT: Mercury-ion optical clock beats cesium standard
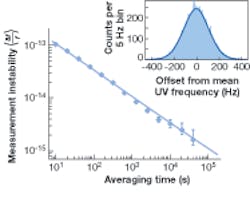
For more than 50 years, the most accurate clocks have been based on the frequency of the cesium atomic ground-state hyperfine transition, with frequency uncertainty values brought down over time to below 10-15. Despite the long-known fact that frequency standards based on an optical transition in a trapped single ion have the potential to achieve uncertainties of 10-18, the optical clocks developed to date have been less reproducible than the cesium standard (because of femtosecond laser-frequency combs and narrow-linewidth lasers)—until now. Researchers at the National Institute of Standards and Technology (NIST; Boulder, CO) report that the uncertainties of the systematic shifts of an optical standard based on the measurement of the absolute frequency of a mercury (Hg) optical clock are smaller than those of the best cesium primary standards.1
The mercury-ion optical clock consists of a 563 nm laser that is doubled and servo-locked to a specific 282 nm electric-quadrupole or “clock” frequency transition in a single laser-cooled 199Hg+ ion held in a cryogenic radio-frequency trap consisting of a ring electrode driven with radio frequencies and two end-cap electrodes held near direct-current ground. A 194 nm laser cools the ion to reduce Doppler shifts and also serves to determine whether the 282 nm laser has driven the ion from the ground state to an excited state. If it is in the ground state, it scatters 194 nm photons, which can be detected by a photomultiplier. If it is in an excited state, it does not scatter 194 nm photons.
Several improvements in systematic uncertainty measurement of the mercury clock transition frequency have led to the precise measurements now being made by the research team. Specifically, errors due to uncertainty of the second-order Zeeman coefficient were reduced by placing the mercury standard in a single-layer magnetic shield and applying a small magnetic field to fix the orientation of the ion. Additional uncertainty due to slow changes in the magnetic field was reduced by periodic measurement of the mercury-ion transition of interest. There is a small shift of the transition frequency due to the electrostatic interaction between the electric quadrupole moment of the ion and electric potential of the trap. The quadrupole shift was basically eliminated by averaging the optical-clock frequencies that were measured for three orthogonal orientations of the magnetic field.
To ensure proper activation of the desired mercury transition and prevent the ion from entering a dark or inactive state due to optical pumping by the 194 nm radiation, the polarization at the light field of the ion was scrambled by radiating the ion with three noncollinear beams with very different frequencies, generated using an acousto-optic modulator.
Correction for altitude difference
In comparing the uncertainty of the mercury optical-frequency standard with the NIST cesium atomic standard, corrections were made for the second-order Zeeman shift and the gravitational redshift due to the altitude difference between the mercury trap and the cesium standard (about 4.5 m). The three magnetic orientations of the mercury standard were averaged to reduce the quadrupole-shift contribution, resulting in the measured fractional uncertainty in the frequency of the mercury optical transition of 5 × 10-17. The addition of other fractional uncertainties in the measurement leads to a total measured uncertainty for the mercury system of less than 7.2 × 10-17, lower than that of the cesium atomic standard.
The researchers next performed a comparison between the measured uncertainties of the mercury optical- and cesium atomic-frequency transitions using a femtosecond-frequency laser comb. This comparison, made possible by the very high accuracy of these two standards, represents the most accurate absolute measurement of an optical frequency to date (see figure). Measurements of optical-transition frequencies of strontium and ytterbium ions by other laboratories have yielded close, although lesser, uncertainty values.
“Mercury is the first of a new generation of optical-frequency standards more reproducible than cesium,” note the researchers. “Eventually one of them will likely replace cesium as the international definition of the second, but right now, it is impossible to predict which one.”
REFERENCE
1. W.H. Oskay et al., Physical Review Letters 97, 020801 (2006).
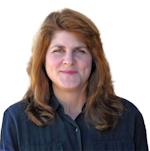
Gail Overton | Senior Editor (2004-2020)
Gail has more than 30 years of engineering, marketing, product management, and editorial experience in the photonics and optical communications industry. Before joining the staff at Laser Focus World in 2004, she held many product management and product marketing roles in the fiber-optics industry, most notably at Hughes (El Segundo, CA), GTE Labs (Waltham, MA), Corning (Corning, NY), Photon Kinetics (Beaverton, OR), and Newport Corporation (Irvine, CA). During her marketing career, Gail published articles in WDM Solutions and Sensors magazine and traveled internationally to conduct product and sales training. Gail received her BS degree in physics, with an emphasis in optics, from San Diego State University in San Diego, CA in May 1986.