PHOTONIC FRONTIERS: SILICON PHOTONICS - Closing in on silicon lasers
Silicon is the foundation of modern semiconductor electronics, and silicon detectors are ubiquitous in optical systems. However, the laws of physics create a formidable roadblock to the “holy grail” of making silicon light-emitters that can be integrated with electronics to interconnect integrated circuits. Silicon crystals have an indirect bandgap structure that makes it difficult for electrons in the conduction band to drop into holes in the valence band and emit light efficiently.
Researchers can’t repeal the laws of physics, but they have found some promising loopholes. One is to form microstructures or nanostructures that alter the energy dynamics of recombination to make light emission more likely. These include porous silicon, etching arrays of holes, or fabricating quantum dots or dislocation loops within the silicon. Another is to turn to stimulated Raman scattering, which was used to demonstrate the first “silicon lasers” in 2004. Other alternatives are to dope the silicon or combine it with elements that enhance radiative efficiency. Developers are making noteworthy strides-two different types of silicon lasers have been reported since late 2004.
The indirect bandgap problem
Silicon’s indirect bandgap doesn’t make light emission impossible, but it does make it very difficult. In a direct-bandgap material like gallium arsenide, electrons can drop from the conduction band into the valence band without changing their momentum. In indirect bandgap materials such as silicon, conduction-band electrons have to change their momentum to drop to the valence band (see Fig. 1). That isn’t impossible, but it takes a while, so the radiative lifetime is on the order of milliseconds.
Some lasers work perfectly well with millisecond radiative lifetimes because the excited species don’t have a faster way to release their energy. However, the electrons and holes in a semiconductor are mobile, and while they’re waiting for radiative decay to happen, they’re likely to encounter defects or trapping centers where they can recombine without emitting light. The nonradiative recombination lifetime in silicon is on the order of nanoseconds, so recombining pairs don’t get a chance to emit light. Other processes also can impede radiative efficiency. The only ways to shift the balance in bulk silicon are to add phonons to shorten the radiative lifetime, or to make the silicon so extremely pure that electrons and holes can travel hundreds of micrometers without encountering a single impurity or flaw. Both have been demonstrated, but neither appears practical.
Porous silicon
The first real hope for light-emitting silicon came from demonstrations of emission from porous silicon produced by chemical etching. By the early 1990s, optical pumping had generated light from porous silicon structures in which chemical etching had left an array of very thin upright rods standing on a silicon substrate. Developers originally thought the rod structures were so small they produced quantum-confinement effects that altered the energy levels of silicon atoms. However, the emission is now generally thought to have been caused by compounds left on the rod surfaces. In addition, porous silicon suffers from high loss and low conductivity. Most research has turned to other ways to produce silicon nanostructures.
Late last year, Jingming Xu’s group at Brown University produced optical gain and stimulated emission by argon-laser pumping of silicon with a different type of internal nanostructure.1 Xu’s group etched 100-nm-scale holes into electronic-grade silicon, leaving a continuous lattice of silicon less than 100 nm thick (see Fig. 2). “The process of drilling has introduced subtle changes to the atomic arrangement between silicon atoms,” Xu says. These changes take place in zones that are 4 or 5 nm thick on the walls of the holes, altering energy levels.When pumping at 514 nm, Xu found emission from the edges of the etched silicon layer at 1260 to 1340 nm, with a sharp peak at 1278 nm. Output power was highest at 10 K, but the sharp peak persisted to 80 K. As the researchers increased pump power, they saw a threshold effect and line narrowing that produced a much sharper spectral peak. High-resolution spectral measurements showed the peak emission wavelength increased with temperature, stepping through a series of five longitudinal modes separated by 96 GHz.
Xu did not include a junction in the structure, noting that this was “to keep the system as clean and as simple as possible” to make a clear case that the emission was intrinsic to the material-and that he had made a silicon laser. His next target is an electrically pumped version, which would require a junction. He says the structure is compatible with conventional silicon integrated circuits. A key limitation so far is the need for cryogenic cooling.
Quantum dots and LEDs
Several groups are working on a different approach to quantum confinement-silicon quantum dots, which can be made as small as 2 nm and are sometimes called nanoparticles. Two properties make them particularly attractive for light-emitting devices. Their quantum confinement of electrons and holes increases their spread in momentum, so the two are more likely to overlap, increasing the chance for radiative recombination. The small size of a quantum dot means that it can contain only on the order of a thousand atoms, greatly reducing the chance of encountering defects that trigger nonradiative recombination as long as surface defects can be avoided.2
Silicon quantum dots can be fabricated in several ways, and generally are dispersed within a matrix of silica or porous silicon (see Fig. 3). Optical pumping readily generates electrons and holes, efficiently producing luminescence. Quantum efficiencies have exceeded 50% in the best experiments. Electrical excitation of quantum dots has yielded light-emitting diodes, but they are far more difficult.3 “There is a rather fundamental conflict between the need for each quantum dot to be electrically separated, so electrons and holes can be confined in the same region, and the need to pass current from one quantum dot to the next,” says Philippe Fauchet of the University of Rochester (Rochester, NY). The key to success is generally the formation of fine silicon threads from the atoms that fail to coalesce into quantum dots, which is not an ideal arrangement. He says quantum wires might work better.Several optical-pumping experiments have yielded evidence for amplified spontaneous emission from quantum dots. At the IEEE International Conference on Group IV Photonics meeting (September 2005; Antwerp, Belgium), Fauchet reported a novel experiment with high-intensity femtosecond-pulse-excited quantum dots embedded in a 0.5-µm surface film on a 100-µm microsphere. Whispering-gallery modes formed a high-Q cavity, and Fauchet says they might have lased, but he has yet to finish all tests.
Other silicon lasers
The first silicon laser, reported in late 2004 by Bahram Jalali of the University of California (Los Angeles, CA) was based on an entirely different process, stimulated Raman scattering of 1540‑nm pump pulses from an erbium-fiber laser. It produced 1675-nm pulses in a 2-cm-long silicon waveguide on a silicon substrate.4 A few months later, Haisheng Rong of Intel (Santa Clara, CA) produced continuous-wave Raman laser action at 1686 nm by pumping at 1550 nm.5 That required sandwiching the S-shaped waveguide, with total length 4.6 cm, in the intrinsic layer of a reverse-biased p-i-n diode to suppress two-photon absorption. However, the silicon Raman laser must be pumped optically, a major drawback because it needs an external pump laser.
Another approach to light emission from silicon is to dope the semiconductor with other elements. Several groups have enhanced electrically pumped light emission by adding erbium to layers containing silicon quantum dots. Excited electron-hole pairs transfer energy to the erbium ions, which then emit near 1535 nm. However, it’s the erbium emitting the light, so it isn’t strictly speaking a silicon emitter.
Outlook
Silicon lasers are part of a broader field of silicon photonics, which also includes modulators and other active devices made from silicon. Bibliographies are growing as researchers push the frontier. And more is coming. Intel, a world leader in silicon electronics, is applying a big push from the commercial side. The U.S. Pentagon has also put silicon-based lasers and nanophotonics on its project list for its Multidisciplinary University Research Initiative. The winners are expected to be announced at the end of this month.
REFERENCES
1. S. Cloutier et al, Nature Materials 4, 887 (Dec. 1, 2005).
2. P. Fauchet, Materials Today 8, 26 (Jan. 2005).
3. L. Pavesi, Materials Today 8, 18 (January 2005).
4. O. Boyraz and B. Jalali, Optics. Exp. 12, 5269 (2004).
5. H. Rong et al, Nature 433, 725 (Feb. 17, 2005).
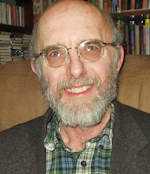
Jeff Hecht | Contributing Editor
Jeff Hecht is a regular contributing editor to Laser Focus World and has been covering the laser industry for 35 years. A prolific book author, Jeff's published works include “Understanding Fiber Optics,” “Understanding Lasers,” “The Laser Guidebook,” and “Beam Weapons: The Next Arms Race.” He also has written books on the histories of lasers and fiber optics, including “City of Light: The Story of Fiber Optics,” and “Beam: The Race to Make the Laser.” Find out more at jeffhecht.com.