NOVEL FIBERS: Co-doped optical fiber serves as gamma-radiation sensor
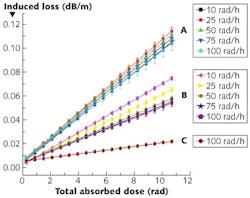
One of the lesser-known qualities of glass is that it can serve as a sensor of gamma radiation. Gamma rays are energetic enough to create color centers, or pointlike defects, in glass; monitoring the optical transmission of the glass is a straightforward way to determine how much gamma radiation it has received. The proportional amount of damage to the glass, and thus the resultant drop in transmission, depends not only on the type of glass but on the dopants in the glass.
Because glass can be used as a radiation detector, its potential benefit to facilities that may have areas of hazardous radiation, such as nuclear-waste storage sites and nuclear reactors, can be great. In the form of an optical fiber routed throughout a facility, glass can serve as a remote distributed radiation sensor that can be easily queried by monitoring its transmission. The challenge is to boost its sensitivity so that relatively low radiation doses can be quantified.
Phosphorus (P)-doped silica (SiO2) fibers have been studied for use in medical radiation dosimeters at high dose rates, and more recently for more general application at lower dose rates down to 1.0 rad/h.1,2 However, when used to measure the total dose over a period of time, these fibers show a strong dependence on the dose rate: In other words, two fibers receiving the same total dose of radiation will produce different results if the length of time over which they receive the dose differs. For safety-related applications in particular, this strong dependence on dose rate is not acceptable.
Step-index multimode profile
A group of scientists from the Central Glass & Ceramic Research Institute–Council of Scientific and Industrial Research (Kolkata, India) and the Defence Laboratory (Jodhpur, India) are experimenting with co-doping silica fibers with germanium dioxide (GeO2) as well as phosphorus pentoxide (P2O5); the doping combination lowers the dose-rate dependency, has a linear response to radiation dose, and has sensitivity high enough to be useful for sensing at nuclear facilities.4
The fiber has a step-index multimode (SIMM) refractive-index profile. For experimentation, the researchers created three different types of fiber: one with a P2O5-doped core (16 mol %), a second with a GeO2-doped core (10 mol %), and a third with a GeO2- and P2O5-doped core (16 and 6 mol %, respectively).
To fabricate the fiber preforms, the various doped mixtures (in the form of soot layers) were deposited via modified chemical vapor deposition (MCVD) on the inside of pure silica tubes with 14 mm outer diameter and 11 mm inner diameter. A single-wavelength online IR pyrometer was used to monitor deposition temperature, which was held to 1500–1550°C. After drawing, the core diameters were 50 μm for the singly doped fibers and 42 μm for the co-doped fiber.
In the test setup, a 4 m length of the test fiber was wrapped around a 2.5-cm-diameter cylinder. Light was coupled into the test fiber from a 100 W quartz-halogen lamp having an output stability of ±0.1% over 8 h. At the fiber’s output end, a silicon photodetector with a wavelength response spanning 400–1100 nm was used for transmission measurement. The cylinder was placed near a cobalt-60 radiation source whose distance was varied to vary the dose rate: Values of 10, 25, 50, 75, and 100 rad/h were used for testing, with a total dose of less than 100 rad (for comparison, the maximum dose received by emergency workers in Japan’s damaged Fukushima nuclear power plant was on the order of 20 rads; the workers spent only brief lengths of time within the plant).
Low sensitivity to dose rate
The fibers’ radiation-induced loss was tested as a function of wavelength, and two wavelengths—505 and 560 nm—selected for experimental use due to their especially high induced loss and high sensitivity. For a total gamma-radiation dose of 100 rad, the radiation sensitivity of the co-doped fiber was found to be 0.69–0.97 dB/m at a 505 nm wavelength. The linearity of the two most-relevant fibers (the co-doped and the P-doped fibers) was measured at dose rates of 10–100 rad/h; both fibers showed a highly linear response (see Fig. 1). In addition, the sensitivity of the co-doped fiber to total radiation dose was almost twice as great as the P-doped fiber, while at the same time the co-doped fiber’s dependence on dose rate was about half that for the P-doped fiber. The combination means that the relative dose-rate sensitivity for co-doped fiber is lower by about a factor of three.
Another quality tested by the researchers was the fibers’ recovery—or in other words, gradual fading of the accumulated absorption after the final dose has been reached. The amount of fading was low even after times of up to an hour after the total dose was reached (see Fig. 2).The researchers hypothesize that the dose-rate sensitivity is reduced because the presence of GeO2 controls the rate of formation of phosphorus-oxygen hole centers (POHCs) as a function of dose rate (POHCs, which form in both stable and metastable versions, are the defect centers formed by the radiation).
REFERENCES
1. P. Liu et al., Can. J. Phys., 78, 89 (2000).
2. E. Regnie et al., IEEE Trans. Nucl. Sci., 54, 1115 (2007).
3. M.C. Paul et al., J. Non-Cryst. Solids, 355, 1496 (2009).
4. Sudipta Ghosh et al., Appl. Opt., 50, 25, E80 (September 2011).
About the Author
John Wallace
Senior Technical Editor (1998-2022)
John Wallace was with Laser Focus World for nearly 25 years, retiring in late June 2022. He obtained a bachelor's degree in mechanical engineering and physics at Rutgers University and a master's in optical engineering at the University of Rochester. Before becoming an editor, John worked as an engineer at RCA, Exxon, Eastman Kodak, and GCA Corporation.